The evolving role of immune oncology in colorectal cancer
Introduction
In the United States colorectal cancer is currently the third most common cancer and worldwide it is the fourth leading cause of cancer related death with an overall lifetime risk of developing the disease estimated to be approximately 4.7% and 4.4% for men and women respectively (1,2). Metastatic colorectal cancer still remains an essentially non curable disease. However, with advances in chemotherapeutic and personalizing therapeutic approaches with the incorporation of targeted therapy such as anti-EGFR and anti-VEGF agents survival associated with metastatic colorectal cancer has certainly improved over the last two decades. However, for those patients not amenable to surgery median survival remains approximately 2 to 3 years. Among patients whose primary and metastatic disease are amenable to resection 5-year survival rates ranging from 26% to 40% have been reported (3).
In an attempt to improve prognostic outcome over the last decade research has focused on therapeutic options geared towards harnessing the immune system. Evidence indicates that the immune system plays a complex role in both the development of cancer and its treatment with significant advances made in our understanding of the relationship that exists between the immune system and cancer (4). This has led to the development of a number of immune therapeutic approaches that has found its way to clinical practice including approaches utilizing cancer vaccine and the use of immunomodulatory monoclonal antibodies (immune checkpoint inhibitors). The use of immune checkpoint inhibitors either as single agent or in combination has certainly become an important part of clinical oncology practice with the FDA approving its use in a variety of advanced malignancies including but not limited to melanoma, lung, kidney, bladder and head and neck cancers. The use of immunomodulatory therapeutic approaches for the management of unselected advanced colorectal cancer has not seen the same success with low response rates reported (4-6). The turning point was our recent understanding that efficacy of immunotherapy was limited to a subset of patients with hyper mutated microsatellite instability-high (MSI-H) tumors. This review will focus on summarizing available knowledge on the immunological and molecular landscape of colorectal cancer that can help determine subsets of immunogenic CRC, determine potential predictive markers to help select patients for immunotherapeutic approaches as well looking at the existing evidence of incorporating immune checkpoint inhibitors to the therapeutic management of subsets of patients with mCRC and the ongoing efforts to increase activity of immunotherapy among patients with non MSI-H tumors through novel combination approaches.
The molecular and immunological landscape of colorectal cancer
Colorectal cancer subtypes
Based on gene expression CRC has been classified into four consensus molecular subtypes (CMS) that although has applications in understanding biology of disease has been used more in the realm of research rather than for routine patient care. CMS1 (MSI-like) comprises 14% of CRC tumors and are characterized by hypermutation, a strong immune activation and includes MSI tumors. CMS2 (canonical) comprises 37% of CRC tumors and consist of chromosomal instable tumors (CIN) with epithelial differential. These tumors also show a strong up-regulation of WNT and MYC downstream targets. CMS3 (metabolic) comprises 13% of CRC tumors that are epithelial and characterized by metabolic dysregulation and includes tumors with KRAS mutations. CMS4 (mesenchymal) include 23% of CRC tumors characterized by a mesenchymal phenotype with prominent transforming growth factor activation, stromal invasion and angiogenesis. A further 13% of CRC tumors exhibit mixed features. A high expression of immune signatures is seen in CMS1 and CMS4 subtypes. CMS1 tumors include those that exhibit expansion or contraction of the microsatellite regions the cause of which is an alteration in the dMMR enzymes associated with an increased mutational rate (due to mutations in the DNA MMR genes) (7). The presence of a high frequency of MSI in CRC has been shown to be associated with a favorable outcome among patients with early stage disease (8). Furthermore MSI-high (MSI-H) tumors have also been shown to be associated with a mutational rate that is 20 times higher than a CRC tumor exhibiting microsatellite stable (MSS) features and as such reflects a tendency to have a higher neoantigen load that can predict for response to immunotherapy (9). CMS4 tumors typically display a high expression of myeloid chemokines, angiogenic factors, immunosuppressive factors and complement components. These factors correlate with worse prognosis in CRC. Therapeutic approaches for this subtype would likely involve an approach that targets the suppressive monocytoid cells and related cytokines, alone or in combination with immune checkpoint inhibition. The classic “cold” tumors are those encompassed in CMS2 and CMS3 subsets and therapeutic approaches for these subsets could potentially include incorporating some form of immunogenic stimulus, such as radiation, a vaccine, or a costimulatory compound.
MSI as a biomarker of response to immunotherapy
As described earlier MSI-H tumors represent a subset of CRC tumors where immunotherapeutic management approaches would be a viable approach. MSI is essentially a marker of dysfunctional mismatch repair proteins (MMR) within a tumor the main function of which is to maintain genomic stability by correcting for single base nucleotide mismatches, insertions, or deletions that can potentially arise during DNA replication (10). Deficiencies in MMR proteins may either be sporadic or be the result of germline mutations. Although 15% of CRC are known to be MSI-H the majority of these cases arise due to an acquired somatic defect in the MMR gene function, most commonly arising secondary to hypermethylation of the MLH1 promoter (11) and less commonly due to two separate mutations that can induce mismatch repair deficiency (12). In approximately 3% of cases, a germline MMR mutation (Lynch Syndrome) is observed and as such patients with MSI-H tumors should be referred for further genetic testing and counselling for Lynch Syndrome (13,14). MSI-H tumors are associated with a favorable prognostic outcome in the early stage setting with data also indicating that adjuvant flurouracil therapy did not improve survival in this cohort in part probably because their risk of relapse was lower than those whose tumors were MSI-Low (MSI-L) (8,15,16). With MSI-H tumors intrinsically associated with a high neoantigen load secondary to a high mutation rate this cohort may be an ideal target for immune therapeutic approaches (9). Early evidence for this came from a phase I trial that was investigating the immune checkpoint inhibitor nivolumab where the investigators observed one patient who attained a complete response that was durable for 21 months (5). The investigators later noted this patient to have an MSI-H CRC tumor with tumor infiltrating macrophages and lymphocytes which were PDL-1+. This finding reinforced the idea that MSI could be a potential biomarker for selecting patients for immunotherapeutic approaches to management. As such reliably testing for this biomarker has also been explored. Testing for tumor MMR deficiency (dMMR) can be done either by directly testing for the expression of MMR proteins (MLH1, MSH2, MSH6 and PMS2) using immunohistochemistry or by MSI testing where by alterations of 5 microsatellite lengths in DNA are assessed using a PCR based methodology with an MSI-H status defined as instability in two or more of the five microsatellites. Studies have reported 92% concordance between the two methodologies (17,18)
Other potential biomarkers of response to immunotherapy
Tumor mutation burden (TMB) and PDL-1 expression have also been explored as potential biomarkers of response to immunotherapy. A high TMB has been associated with a higher probability of response to immune checkpoint inhibitors secondary to an associated higher neoantigen load that is recognized as foreign by the host (19,20). An example includes patients with MSI-H CRC that is known to be associated with high TMB (9). Mutations in the genes encoding for DNA polymerase d (POLD1) and e (POLE) have also been shown to be associated with a hypermutated phenotype that would likely also respond to immune therapeutic approaches (21,22).
The predictive value of PD-L1 expression as a potential biomarker of response has been explored in a number of studies, however results have been conflicting depending on the tumor type explored and type of antibody used to test for PD-L1 expression. Carbognin and colleagues conducted a meta-analysis of 20 phase I–III trials that looked at immune checkpoint inhibitors in the treatment of advanced melanoma, non-small lung cancer and genitourinary cancer (23). The objective of the analysis was to look at the differential activity of immune checkpoint inhibitors in solid tumors based on PDL-1 expression. The authors reported overall response rates of 34.1% and 19.9% in PD-L1–positive and PD-L1–negative tumors, respectively. In the realm of CRC emerging data suggests that PDL-1 expression may not have the same predictive value. In the Keynote-028 trial one out of twenty three patients with PDL-1 expressing mCRC demonstrated a response to pembrolizumab with the responding patient later on determined to have an MSI-H tumor (24). Overmann and colleagues observed that among patients with MSI-H mCRC treated on the Checkmate-142 trial with nivolumab responses were observed irrespective of the PDL-1 expression (25).
The immunoscore
Accumulating data indicates that the presence of tumor infiltrating lymphocytes (defined asCD3+and CD45RO+ cells within a tumor) is known to be associated with patient prognostic outcome (26). Longer progression-free and overall survival (OS) has been shown to be associated with presence of infiltrating memory T cells and CD8+ T cells (27). Such observations led to the development of the immunoscore which attempts to define and quantify the in situ immune infiltrate (28). The immunoscore is calculated based on counting of two lymphocyte populations (defined as CD3/CD45RO, CD3/CD8 or CD8/CD45RO positivity) in both the tumor core and invasive margin and then coming up with a score ranging from 0 (where you observe a low density of both cells in both regions) to a score of 4 (defined has high density of both cells) with each score attached to a predictive value enabling identification of patients with early stage disease who might benefit from adjuvant therapy (28,29). An analysis of 270 patients derived from The Cancer Genome Atlas revealed a higher intratumor gene expression and high immunoscore among patients with MSI-H tumors compared to those with MSS tumors supporting the use of immune therapeutic regimens among patients with MSI-H tumors (30,31).
Collectively the use and integration of the various biomarkers discussed so far may help further characterize and define subsets of patients who would have a high probability of responding to immunotherapy among patients who have MSS tumors and potentially identify those patients with MSI-H tumors who may not respond to immunotherapy.
Check point inhibition: alone and in combination
The function of immune checkpoints is to downregulate immune responses after neoantigen activation and as such tumor cells that express immune checkpoints including PD-1 and CTL-4 are able to evade the immune system (6). Over the last decade inhibition of PD-1 and CTL-4 with monoclonal antibodies had changed the landscape of cancer therapeutics for a variety of malignancies including melanoma, non-small cell lung cancer, renal cell carcinoma and Hodgkins lymphoma (32). Despite these encouraging results the use of immune checkpoint inhibitors in the therapeutic management of CRC has not shown the same benefit across the whole cohort but has been limited to subsets of patients with MSI-H tumors.
In one of the earlier phase I trials Brahmer and colleagues looked at the use of the anti-PD-1 immune checkpoint inhibitor nivolumab among 39 patients with advanced solid tumors (33). The authors observed that among the 14 patients with refractory mCRC one achieved a complete response and that the tumor of this patient was MSI-H with a high number of T cell and increased expression of PD-L1. This patient was reported on long term follow up to have a sustained CR for more than 3 years. In a phase Ib trial evaluating 296 patients with advanced solid tumors receiving single agent nivolumab Topalian and colleagues reported no objective response among the 19 patients who had mCRC (6).
In an attempt to further explore the use of immune checkpoint inhibitors among patients with mCRC likely to achieve a response two trials were initiated among patients who had MSI-H tumors (Table 1). In the phase II Keynote-164 trial Le and colleagues looked at the efficacy of pembrolizumab among patients with progressive metastatic carcinoma with or without dMMR. The trial enrolled patients with dMMR and proficient(p) MMR mCRC as well as patients with dMMR advanced cancers that were not CRC (34,38). A number of interesting observations were made. First, whole exome sequencing not surprisingly revealed 1,782 and 73 somatic mutations per tumor in the dMMR and pMMR cohorts respectively (P=0.007). Furthermore, the authors observed a correlation between prolongation of progression free survival (PFS) and high somatic mutation load (P=0.02). Second, the authors reported an objective response rate (ORR) of 52% and 0% among patients with dMMR and pMMR mCRC respectively. Median PFS and OS was not reached for the MMR-D cohort and was 2.2 and 5.0 months respectively for the pMMR cohort. Third, a further post hoc comparison comparing the two cohorts revealed a HR for progression or death was 0.10 (P<0.001) and a HR for death being 0.22 (P=0.05) favoring the group who had dMMR tumors. Among the 46 patients with dMMR non CRC solid tumors the authors reported an ORR of 54% and a median PFS of 18.4 months. Median OS was not reached in this cohort. Diaz and colleagues recently reported on the combined analysis of patients with MSI-H tumors enrolled on two global, multicenter phase II trials (Keynote164 and 158) (39). At a median follow up of 7.4 months for patients with MSI-H mCRC and 4.5 months for MSI-H non CRC patients the authors reported an ORR and disease control rate (DCR) of 26.2% and 50.8% respectively among patients with CRC and 42.9% and 66.7% respectively among patients with non CRC tumors. Median duration of response for both cohorts was not reached and 100% of responses were still ongoing at the time of reporting the data.
The second trial looked at the use of the immune checkpoint inhibitor nivolumab in the CheckMate 142 trial (25) (Table 1). In this ongoing open label phase II trial Overmann and colleagues reported on 74 patients with dMMR mCRC who had received at least one prior line of therapy. At a median follow up of 12 months the authors reported an ORR of 31.1% and a DCR at 12 weeks of 69% with median duration of response not reached. In a more recent update the authors reported outcome stratified by whether patients received three or more lines of therapy (40). The authors reported an overall median PFS of 6.6 months for the whole cohort. Median PFS was 4.2 months amongst those patients who had received >3 lines of therapy compared to not reached amongst those who had received less lines of prior therapy. Similarly 12 months OS was 68% and 81% amongst those who did and did not receive >3 lines of therapy. The increased robustness of response in earlier line settings has generated immense interest in exploring immune checkpoint inhibitors in first line setting of patients with dMMR mCRC. The investigators of the CheckMate 142 trial also looked at potential predictive biomarkers to response to nivolumab. No difference in response to nivolumab was observed when stratified by PDL-1 expression (≥1% vs. <1%) and lynch syndrome (vs. sporadic). Furthermore no differential response was observed based on presence or absence of KRAS and BRAF mutations (25). This was an especially important observation since the presence of BRAF V600E mutation in a cohort of patients whose tumors are also pMMR tends to be associated with more aggressive disease and lower clinical response to chemotherapy while its presence in a cohort whose tumors are dMMR tend to have a poorer prognosis (41).
Based on the results of the phase II trials the Food and Drug administration on the 23rd of May 2017 approved single agent pembrolizumab for the treatment of adult and pediatric patients with advanced MSI-H or dMMR solid tumors regardless of the tumor site or histology (42). This was the first FDA approval agnostic of cancer type. On the 31st of July 2017 the FDA then granted accelerated approval of single agent nivolumab for patients with mCRC tumors harboring MSI-H or dMMR phenotype (43). For both drugs, patients with mCRC had to have prior therapies that were standard of care.
In an effort to improve response rates and make tumors more immunogenic, combination strategies have been explored (Table 1). Overmann and colleagues reported on a second cohort of 119 patients with dMMR/MSI-H mCRC tumors who were treated with a combination of nivolumab (3 mg/kg) and the anti-CTL-4 immune checkpoint inhibitor ipilimumab (1 mg/kg) in the CheckMate 142 trial (35). Patients needed to have progressed or were intolerant to one or more lines of standard therapy. The combination strategy was given every 3 weeks and for 4 doses and then followed by single agent nivolumab every 2 weeks. At a median follow up of 13.4 months the authors reported ORR and DCR of 55% and 80% respectively. Seventy-eight percent of evaluable patients had a reduction in tumor burden from baseline and median time response was 2.8 months (range, 1 to 14 months) with 83% of responses observed lasting >6 months. PFS and at 12 months was 71% and 85% respectively. At the time of reporting median PFS and OS were not reached. Similar to the single agent cohort, responses were observed irrespective of PDL-1 expression, BRAF and KRAS status and the presence or absence of Lynch syndrome. Although the results appear slightly improved compared to single agent nivolumab the two cohorts enrolled in the CheckMate 142 trial were not randomized and thus direct comparisons are difficult.
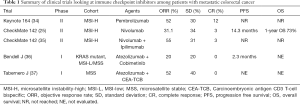
Full table
Immune checkpoint inhibitors have also been explored in combination with chemotherapy and with bevacizumab. With bevacizumab known to play an important role in lymphocyte tracking and immune regulation Hochester and colleagues reported on a novel combination of the immune checkpoint inhibitor atezolizumab and bevacizumab (44). The authors observed that among 10 patients with MSI-H tumors who were enrolled, 70% of whom had received prior bevacizumab and 30% had Lynch syndrome, ORR and a DCR of 40% and 90% respectively was observed. Bendell and colleagues reported on a combination of FOLFOX, bevacizumab and atezolizumab (45). Among 23 patients who were treatment naïve the authors observed an ORR of 87% and a partial response of 48%.
The use of radiotherapy and thermal therapy has been thought to make tumors more immunogenic and this concept has been taken forward in studies looking at these modalities in combination with immune checkpoint inhibitors. Duffy and colleagues looked at the combination of an immune checkpoint inhibitors (AMP224) in combination with liver directed stereotactic body radiation therapy (SBRT) with no safety signals demonstrated (46).
The MAP kinase pathway is one of the most frequently dysregulated pathways in cancer. Dysregulation of this pathway results in sustained inhibition of RAS, RAF, MEK and ERK signaling that ultimately results in uncontrolled proliferation. MEK inhibitors may be one way to stop this pathway. Cobimetinib is a highly selective, potent and reversible inhibitor of MEK1 and MEK2. Single agent cobimetinib has been approved in the treatment of melanoma but has shown little activity among patients with mCRC (47). Pre-clinical data indicate that the combination of atezolizumab and cobimetinib results in tumor regression (48). In a phase Ib dose escalation and cohort expansion study Bendell and colleagues explored this combination in a cohort of patients with advanced solid tumors (36). The authors reported that among 20 patients with KRAS mutant chemo refractory mcRC the ORR and partial response was 20% with a median time to response of 3.7 months. Among patients enrolled 30% had MSS or MSI—low tumors, 0% had MSI-H tumors with the MSI status being unknown for 70% of patients. Among the 4 patients who had a partial response 3 of the responders were MSI-H with the MSI status of the fourth responder not being evaluable. These results represent an improvement over the negligible ORR that is typically seen in this cohort with immune checkpoint inhibitors. The authors further reported a median and 6-month PFS of 2.3 months and 39% respectively and a median and 6-month OS was not reached and 77% respectively. Based on these encouraging early results the expansion of this phase Ib trial is ongoing and a phase III trial is evaluating this combination among patients with chemotherapy refractory mCRC.
Carcinoembryonic antigen CD3 T-cell bispecific (CEA-TCB) antibody is a novel T-cell bispecific antibody that simultaneously binds to tumor and T cells, engages and activates T cells and increases T-cell activation (49). Over 90% of mCRC expresses high levels of CEA making CEA-TCB an idea agent to be investigated in this cohort (50). Preclinical models demonstrated potent anti-tumor activity, increased intra-tumoral T cell infiltration, activation and up regulation of PD-1/PDL-1 and enhanced activity when combined with atezolizumab (51). Tabernero and colleagues recently reported on the efficacy and safety of CEA-TCB either as a single agent or in combination with atezolizumab in cohort of patients with mCRC enrolled in two ongoing phase I trials (37). The majority of patients had MSS disease. Among the 31 patients who received single agent CEA-TCB partial response and DCR was 6% and 45% respectively. Among the 25 patients treated with a combination of CEA-TCB and atezolizumab partial response and DCR was 12% and 52% respectively.
Adverse events related with immune checkpoint inhibitors
The use of immune checkpoint inhibitors among patients with CRC has not resulted in any specific side effects different from that reported in clinical trials across solid tumors (25). Commonly encountered side effects include skin toxicity (such as rash and puriitis) that occurs in approximately >20% of patients, fatigue, infections and peripheral edema. Other side effects reported in less than 10% of cases include arrhythmias, infusion-related reactions, iridocyclitis and peripheral and sensory neuropathy. Laboratory related abnormalities include but are not limited to disturbances in liver enzymes, hyponatremia, hyperkalemia, disturbances in thyroid function and changes in cortisol levels (52).
Going beyond check point inhibition
Another method of modulating the immune system is the use of therapeutic cancer vaccines that facilitates immune destruction of cancer cells by helping to activate and maintain an anti-tumor response. Using cancer vaccines has the potential to induce tumor specific cytotoxic T cells and humoral responses to tumor antigens. Unfortunately, despite the promise of such as strategy objective clinical activity has been limited. This is likely due to the development tolerance to self-antigens. In the realm of CRC the use of whole-cell, peptide, virus-based and dendritic cell vaccines have been investigated. Rao and colleagues conducted a meta-analysis of clinical trials looking at vaccine therapies in CRC (53). The authors reported a benefit in OS and disease free survival with the use of vaccine therapies in the adjuvant setting with little benefit observed in the advanced setting. Potential methods that are being explored to overcome tolerance mechanisms that develop with the use of cancer vaccines include combinations with checkpoint inhibitors and refining methods to more specifically predict individual patient tumor neoantigens that can eventually result in the development of patient-specific personalized vaccines (54,55).
Another promising strategy explored to enhance tumor-specific adaptive immune response has been the use of adoptive T-cell transfer which involves transferring modified including genetically engineered immune cells to the host. The most promising strategy of adoptive T–cell transfer has been the use of chimeric antigen receptor (CAR) T-cell therapy. CAR-T cell therapy uses autologous T cells that have been engineered to express tumor-specific receptors. The use of CAR-T cell therapy has seen significant success in the treatment of hematological B-cell malignancies highlighted by the recent FDA approval of CAR-T cell therapy for patients with acute lymphoblastic leukemia (56). In the realm of solid tumors, the role of CAR-T cells has yet to show significant clinical impact. In a phase I trial, Parkhurst and colleagues treated three patients with chemotherapy-refractory mCRC with autologous T cells that that had been genetically modified to express a murine T cell receptor against CEA (57). The authors reported significant reduction in the CEA levels of all three patients with one patient attaining an objective clinical response. Unfortunately, all three patients developed severe transient colitis that resulted halting of study accrual. In a phase I/II trial Zhen and colleagues reported on 55 patients (stage I–IV CRC) who underwent radical surgery where intraoperative sentinel lymph node was identified (58). Sentinel lymph node T lymphocytes were then expanded ex vivo and there after transfused. The authors reported no treatment related toxicity. The 2-year survival rate of the group receiving the sentinel lymph node T-cells 55.6% versus 17.5% for the control group (P=0.02). Tran and colleagues reported on a patient that was enrolled in a phase II trial that looked specifically at whether regression of metastatic solid cancers could be achieved with adoptive transfer of ex vivo expanded tumor infiltrating lymphocytes (59). The case reported was of a patient with KRAS G12D mutant mCRC with 10 lung metastatic lesions. The authors observed objective regression of 7 lesions in the lungs after a single infusion of tumor infiltrating lymphocytes that consisted of CD8+T cells that were reactive to mutant KRASG12D.
Conclusions
The long term prognosis of patients with mCRC remains poor despite efforts to develop novel chemotherapeutic and targeted regimens. The fundamental understanding that CRC is not one disease but a conglomerate of a number of important subtypes is likely to guide future therapeutic management and ultimately improve prognostic outcome. RAS wild type tumors benefit from anti-EGFR therapy especially in left-sided colorectal cancers, tumors that over express HER2 may benefit from anti-HER2 therapy and BRAF mutated tumors are known to be inherently resistant to chemotherapy. Although the use of immune checkpoint inhibitors has resulted in significant success in the management of solid tumors such melanoma, lung cancer and kidney cancer it has seen limited success when used in an unselected CRC cohort. Its use and phenomenal success in the subset of patients with MSI-H mCRC where immune checkpoint inhibitors have significantly improved survival in a chemorefractory cohort known to traditionally have a poor prognostic outcome and the recognition that this success could be replicated across MSI-H solid tumor types highlights that certain biomarkers are agnostic of tumor type. Future research in the realm of CRC with immune therapeutic approaches will now likely focus on taking immune checkpoint inhibitors to earlier line settings as well as identifying approaches to improve immunogenicity of non MSI-H CRC. Data on combination strategies of immune checkpoint inhibitors with MEK inhibitors and CEA-TCB among patients with non MSI-H CRC are certainly encouraging and are actively being investigated. The future ahead is certainly exciting and it is no wonder that the American Society of Clinical Oncology (ASCO) declared Immunotherapy to be the clinical advance of the year in 2016 with Immunotherapy 2.0 being the advance of 2017.
Acknowledgements
None.
Footnote
Conflicts of Interest: The author has no conflicts of interest to declare.
References
- Siegel RL, Miller KD, Fedewa SA, et al. Colorectal cancer statistics, 2017. CA Cancer J Clin 2017;67:177-93. [Crossref] [PubMed]
- Arnold M, Sierra MS, Laversanne M, et al. Global patterns and trends in colorectal cancer incidence and mortality. Gut 2017;66:683-91. [Crossref] [PubMed]
- McLoughlin JM, Jensen EH, Malafa M. Resection of colorectal liver metastases: current perspectives. Cancer Control 2006;13:32-41. [Crossref] [PubMed]
- Markman JL, Shiao SL. Impact of the immune system and immunotherapy in colorectal cancer. J Gastrointest Oncol 2015;6:208-23. [PubMed]
- Brahmer JR, Tykodi SS, Chow LQ, et al. Safety and activity of anti-PD-L1 antibody in patients with advanced cancer. N Engl J Med 2012;366:2455-65. [Crossref] [PubMed]
- Topalian SL, Hodi FS, Brahmer JR, et al. Safety, activity, and immune correlates of anti-PD-1 antibody in cancer. N Engl J Med 2012;366:2443-54. [Crossref] [PubMed]
- Guinney J, Dienstmann R, Wang X, et al. The consensus molecular subtypes of colorectal cancer. Nat Med 2015;21:1350-6. [Crossref] [PubMed]
- Lynch HT, Snyder CL, Shaw TG, et al. Milestones of Lynch syndrome: 1895-2015. Nat Rev Cancer 2015;15:181-94. [Crossref] [PubMed]
- Gryfe R, Kim H, Hsieh ET, et al. Tumor microsatellite instability and clinical outcome in young patients with colorectal cancer. N Engl J Med 2000;342:69-77. [Crossref] [PubMed]
- Kocarnik JM, Shiovitz S, Phipps AI. Molecular phenotypes of colorectal cancer and potential clinical applications. Gastroenterol Rep (Oxf) 2015;3:269-76. [PubMed]
- Modrich P. Mechanisms in eukaryotic mismatch repair. J Biol Chem 2006;281:30305-9. [Crossref] [PubMed]
- Poynter JN, Siegmund KD, Weisenberger DJ, et al. Molecular characterization of MSI-H colorectal cancer by MLHI promoter methylation, immunohistochemistry, and mismatch repair germline mutation screening. Cancer Epidemiol Biomarkers Prev 2008;17:3208-15. [Crossref] [PubMed]
- Haraldsdottir S, Hampel H, Tomsic J, et al. Colon and endometrial cancers with mismatch repair deficiency can arise from somatic, rather than germline, mutations. Gastroenterology 2014;147:1308-16.e1. [Crossref] [PubMed]
- Jenkins MA, Hayashi S, O'Shea AM, et al. Pathology features in Bethesda guidelines predict colorectal cancer microsatellite instability: a population-based study. Gastroenterology 2007;133:48-56. [Crossref] [PubMed]
- Yurgelun MB, Kulke MH, Fuchs CS, et al. Cancer Susceptibility Gene Mutations in Individuals With Colorectal Cancer. J Clin Oncol 2017;35:1086-95. [Crossref] [PubMed]
- Ribic CM, Sargent DJ, Moore MJ, et al. Tumor microsatellite-instability status as a predictor of benefit from fluorouracil-based adjuvant chemotherapy for colon cancer. N Engl J Med 2003;349:247-57. [Crossref] [PubMed]
- Shia J. Immunohistochemistry versus microsatellite instability testing for screening colorectal cancer patients at risk for hereditary nonpolyposis colorectal cancer syndrome. Part I. The utility of immunohistochemistry. J Mol Diagn 2008;10:293-300. [Crossref] [PubMed]
- Shia J, Stadler Z, Weiser MR, et al. Immunohistochemical staining for DNA mismatch repair proteins in intestinal tract carcinoma: how reliable are biopsy samples? Am J Surg Pathol 2011;35:447-54. [Crossref] [PubMed]
- Rizvi NA, Hellmann MD, Snyder A, et al. Cancer immunology. Mutational landscape determines sensitivity to PD-1 blockade in non-small cell lung cancer. Science 2015;348:124-8. [Crossref] [PubMed]
- Snyder A, Makarov V, Merghoub T, et al. Genetic basis for clinical response to CTLA-4 blockade in melanoma. N Engl J Med 2014;371:2189-99. [Crossref] [PubMed]
- Kothari N, Teer JK, Abbott AM, et al. Increased incidence of FBXW7 and POLE proofreading domain mutations in young adult colorectal cancers. Cancer 2016;122:2828-35. [Crossref] [PubMed]
- Ahn SM, Ansari AA, Kim J, et al. The somatic POLE P286R mutation defines a unique subclass of colorectal cancer featuring hypermutation, representing a potential genomic biomarker for immunotherapy. Oncotarget 2016;7:68638-49. [Crossref] [PubMed]
- Carbognin L, Pilotto S, Milella M, et al. Differential Activity of Nivolumab, Pembrolizumab and MPDL3280A according to the Tumor Expression of Programmed Death-Ligand-1 (PD-L1): Sensitivity Analysis of Trials in Melanoma, Lung and Genitourinary Cancers. PLoS One 2015;10:e0130142. [Crossref] [PubMed]
- O’Neil BH, Wallmark J, Lorente D, et al. Pembrolizumab (MK-3475) for patients (pts) with advanced colorectal carcinoma (CRC): Preliminary results from KEYNOTE-028. Eur J Cancer 2015;51:abstr s103.
- Overman MJ, McDermott R, Leach JL, et al. Nivolumab in patients with metastatic DNA mismatch repair-deficient or microsatellite instability-high colorectal cancer (CheckMate 142): an open-label, multicentre, phase 2 study. Lancet Oncol 2017;18:1182-91. [Crossref] [PubMed]
- Becht E, Giraldo NA, Germain C, et al. Immune Contexture, Immunoscore, and Malignant Cell Molecular Subgroups for Prognostic and Theranostic Classifications of Cancers. Adv Immunol 2016;130:95-190. [Crossref] [PubMed]
- Becht E, de Reyniès A, Giraldo NA, et al. Immune and Stromal Classification of Colorectal Cancer Is Associated with Molecular Subtypes and Relevant for Precision Immunotherapy. Clin Cancer Res 2016;22:4057-66. [Crossref] [PubMed]
- Galon J, Mlecnik B, Bindea G, et al. Towards the introduction of the 'Immunoscore' in the classification of malignant tumours. J Pathol 2014;232:199-209. [Crossref] [PubMed]
- Mlecnik B, Bindea G, Kirilovsky A, et al. The tumor microenvironment and Immunoscore are critical determinants of dissemination to distant metastasis. Sci Transl Med 2016;8:327ra26. [Crossref] [PubMed]
- Mlecnik B, Bindea G, Angell HK, et al. Integrative Analyses of Colorectal Cancer Show Immunoscore Is a Stronger Predictor of Patient Survival Than Microsatellite Instability. Immunity 2016;44:698-711. [Crossref] [PubMed]
- Inaguma S, Lasota J, Wang Z, et al. Clinicopathologic profile, immunophenotype, and genotype of CD274 (PD-L1)-positive colorectal carcinomas. Mod Pathol 2017;30:278-85. [Crossref] [PubMed]
- Mellman I, Coukos G, Dranoff G. Cancer immunotherapy comes of age. Nature 2011;480:480-9. [Crossref] [PubMed]
- Brahmer JR, Drake CG, Wollner I, et al. Phase I study of single-agent anti-programmed death-1 (MDX-1106) in refractory solid tumors: safety, clinical activity, pharmacodynamics, and immunologic correlates. J Clin Oncol 2010;28:3167-75. [Crossref] [PubMed]
- Le DT, Uram JN, Wang H, et al. PD-1 Blockade in Tumors with Mismatch-Repair Deficiency. N Engl J Med 2015;372:2509-20. [Crossref] [PubMed]
- Overman MJ, Lonardi S, Wong KYM, et al. Durable Clinical Benefit With Nivolumab Plus Ipilimumab in DNA Mismatch Repair-Deficient/Microsatellite Instability-High Metastatic Colorectal Cancer. J Clin Oncol 2018;36:773-9. [Crossref] [PubMed]
- Bendell JC, Kim TW, Goh BC, et al. Clinical activity and safety of cobimetinib (cobi) and atezolizumab in colorectal cancer (CRC). J Clin Oncol 2016;34:3502. [PubMed]
- Tabernero J, Melero I, Ros W, et al. Phase Ia and Ib studies of the novel carcinoembryonic antigen (CEA) T-cell bispecific (CEA CD3 TCB) antibody as a single agent and in combination with atezolizumab: Preliminary efficacy and safety in patients with metastatic colorectal cancer (mCRC). J Clin Oncol 2017;35:3002. [PubMed]
- Le DT, Durham JN, Smith KN, et al. Mismatch repair deficiency predicts response of solid tumors to PD-1 blockade. Science 2017;357:409-13. [Crossref] [PubMed]
- Diaz LA, Marabelle A, Delord JP, et al. Pembrolizumab therapy for microsatellite instability high (MSI-H) colorectal cancer (CRC) and non-CRC. J Clin Oncol 2017;35:3071.
- Overman MJ, Bergamo F, Mcdermott RS, et al. Nivolumab in patients with DNA mismatch repair-deficient/microsatellite instability-high (dMMR/MSI-H) metastatic colorectal cancer (mCRC): long-term survival according to prior line of treatment from CheckMate-142. J Clin Oncol 2018;36:abstr 554.
- Venderbosch S, Nagtegaal ID, Maughan TS, et al. Mismatch repair status and BRAF mutation status in metastatic colorectal cancer patients: a pooled analysis of the CAIRO, CAIRO2, COIN, and FOCUS studies. Clin Cancer Res 2014;20:5322-30. [Crossref] [PubMed]
- Lemery S, Keegan P, Pazdur R. First FDA Approval Agnostic of Cancer Site - When a Biomarker Defines the Indication. N Engl J Med 2017;377:1409-12. [Crossref] [PubMed]
- FDA grants nivolumab accelerated approval for MSI-H or dMMR colorectal cancer. Available online: www.fda.gov/Drugs/InformationOnDrugs/ApprovedDrugs/ucm569366.htm
- Hochster HS, Bendell JC, Cleary JM, et al. Efficacy and safety of atezolizumab (atezo) and bevacizumab (bev) in a phase Ib study of microsatellite instability (MSI)-high metastatic colorectal cancer (mCRC). J Clin Oncol 2017;35:673. [Crossref]
- Bendell JC, Powderly JD, Lieu CH, et al. Safety and efficacy of MPDL3280A (anti-PDL1) in combination with bevacizumab (bev) and/or FOLFOX in patients (pts) with metastatic colorectal cancer (mCRC). J Clin Oncol 2015;33:704. [Crossref]
- Duffy AG, Makarova-Rusher OV, Fioravanti S, et al. A pilot study of AMP-224, a PD-L2 Fc fusion protein, in combination with stereotactic body radiation therapy (SBRT) in patients with metastatic colorectal cancer. J Clin Oncol 2016;34:560. [Crossref]
- Dhillon AS, Hagan S, Rath O, et al. MAP kinase signalling pathways in cancer. Oncogene 2007;26:3279-90. [Crossref] [PubMed]
- Ebert PJR, Cheung J, Yang Y, et al. MAP Kinase Inhibition Promotes T Cell and Anti-tumor Activity in Combination with PD-L1 Checkpoint Blockade. Immunity 2016;44:609-21. [Crossref] [PubMed]
- Bacac M, Fauti T, Sam J, et al. A Novel Carcinoembryonic Antigen T-Cell Bispecific Antibody (CEA TCB) for the Treatment of Solid Tumors. Clin Cancer Res 2016;22:3286-97. [Crossref] [PubMed]
- Tiernan JP, Perry SL, Verghese ET, et al. Carcinoembryonic antigen is the preferred biomarker for in vivo colorectal cancer targeting. Br J Cancer 2013;108:662-7. [Crossref] [PubMed]
- Lehmann S, Perera R, Grimm HP, et al. In Vivo Fluorescence Imaging of the Activity of CEA TCB, a Novel T-Cell Bispecific Antibody, Reveals Highly Specific Tumor Targeting and Fast Induction of T-Cell-Mediated Tumor Killing. Clin Cancer Res 2016;22:4417-27. [Crossref] [PubMed]
- Center for drug evaluation and research. Available online: https://www.accessdata.fda.gov/drugsatfda_docs/nda/2014/125554Orig1s000SumR.pdf
- Rao B, Han M, Wang L, et al. Clinical outcomes of active specific immunotherapy in advanced colorectal cancer and suspected minimal residual colorectal cancer: a meta-analysis and system review. J Transl Med 2011;9:17. [Crossref] [PubMed]
- Schumacher TN, Schreiber RD. Neoantigens in cancer immunotherapy. Science 2015;348:69-74. [Crossref] [PubMed]
- Zhang X, Sharma PK, Peter Goedegebuure S, et al. Personalized cancer vaccines: Targeting the cancer mutanome. Vaccine 2017;35:1094-100. [Crossref] [PubMed]
- FDA D.I.S.C.O.: First FDA approval of a CAR T-cell immunotherapy. Available online: https://www.fda.gov/Drugs/InformationOnDrugs/ApprovedDrugs/ucm598029.htm
- Parkhurst MR, Yang JC, Langan RC, et al. T cells targeting carcinoembryonic antigen can mediate regression of metastatic colorectal cancer but induce severe transient colitis. Mol Ther 2011;19:620-6. [Crossref] [PubMed]
- Zhen YH, Liu XH, Yang Y, et al. Phase I/II study of adjuvant immunotherapy with sentinel lymph node T lymphocytes in patients with colorectal cancer. Cancer Immunol Immunother 2015;64:1083-93. [Crossref] [PubMed]
- Tran E, Robbins PF, Lu YC, et al. T-Cell Transfer Therapy Targeting Mutant KRAS in Cancer. N Engl J Med 2016;375:2255-62. [Crossref] [PubMed]