Emerging role of immunotherapy for childhood cancers
Introduction
While adjuvant or neoadjuvant chemotherapy, surgery and radiation are still the major pillars in the treatment of pediatric malignancies, tremendous progress has been made in the treatment of patients who are either refractory or who have relapsed after current standard therapies. Recent developments have fundamentally changed immunotherapeutic approaches to adult patients with cancer, but also to pediatric malignancies. In contrast to adult cancers, the most frequent malignancy in children is acute leukemias, followed by brain tumors, lymphomas, neuroblastoma and various forms of sarcomas. Here, we will review successes, challenges and future perspectives of different immunotherapeutic approaches to the main pediatric malignancies
Hematological malignancies
Acute lymphoblastic leukemia (ALL)
Bispecific anti-CD3/anti-CD19 T-cell engager blinatumomab for B-cell precursor ALL
A major breakthrough in the treatment of refractory B-lineage leukemias was the engineering and clinical application of the single-chain bispecific antibody construct, also named bispecific T-cell engagers (BiTE) (1). The BiTE format consists of two single-chain variable fragments specific for CD3 (expressed on T lymphocytes) and a tumor antigen. Since CD19 is expressed on almost all B-lineage lymphoblastic leukemias, a CD19-specific BiTE has been engineered and has been introduced into the treatment of CD19-positive hematological malignancies (2). The first clinical application of such an anti-CD3-anti-CD19 BiTE (originally named MT103 and subsequently changed to blinatumomab) was reported in adult patients with CD19-positive lymphomas (3). In this study, an overall response rate of 69% and a median response duration of 13.5 months was demonstrated.
The first three pediatric patients with refractory ALL who received Blinatumomab were treated under a compassionate use approval (4). The first patient was treated at the Children’s University Hospital in Tuebingen, Germany. This was a 7-year-old boy diagnosed in 2004 with high-risk B-precursor ALL. After standard chemotherapy for high risk ALL, he experienced a bone marrow relapse. After multiple cycles of chemotherapies, he achieved a second complete remission (CR) and underwent an allogeneic bone marrow transplantation from 9 out of 10 matched unrelated donor. One year post-transplant, he experienced another relapse. Despite multiple cycles of chemotherapy, the patient had persistence of his leukemia. After regulatory approval of a compassionate use application of the CD3xCD19 BiTE, the patient was then treated with MT103 at 15 µg/m2/day for 5 weeks by continuous intravenous infusion. After 2 weeks of treatment, a bone marrow aspiration showed a minimal residual disease (MRD)-negative remission. Two weeks after end of the BiTE treatment, he underwent a haploidentical T-cell depleted stem cell transplantation and as of February 2018, the patient is in continuous CR (own unpublished result). The second patient was treated at the Children’s Hospital of the Charite, Berlin, Germany. This patient had a previous allogeneic bone marrow transplant with a post-transplant relapse. He responded to chemotherapy, but had subsequently a third relapse, which was refractory to a variety of chemotherapeutic agents. At start of 15 µg/m2/day of blinatumomab, he had 90% infiltration of his bone marrow and 23% peripheral blasts. After one day of blinatumomab, the peripheral blood was cleared from blasts, as measured by flow cytometry. The patient became aplastic until day 15 after start of blinatumomab and subsequently showed a complete recovery of donor-derived hematopoiesis. At day 42, he had a normal hematopoiesis and a MRD-negative CR was documented. Unfortunately, the patient was not eligible for a second stem cell transplantation and relapsed a few weeks later. The third patient had a Philadelphia chromosome-positive B-precursor ALL diagnosed in 2001. This patient had multiple relapses and had received two allogeneic bone marrow transplantations from a human leukocyte antigen (HLA)-identical sibling and an HLA-identical matched unrelated donor, respectively. After the third transplant, the patient remained MRD-positive and received a total of four 4-week courses of 15 µg/m2/day blinatumomab. After this treatment, a bone marrow aspiration showed a MRD-negative CR. However, few weeks later, the patient experienced a relapse in the central nervous system (CNS) and was treated by intrathecal chemotherapy. This clinical observation was an indication, that blinatumomab might not be able to penetrate the CNS at least at the currently used doses. As of February 2018, the patient has another combined BM/CNS relapse and is awaiting experimental therapy.
Besides the impressive MRD-negative responses in these very chemotherapy-refractory patients, it was remarkable that none of the patients developed acute nor chronic graft-versus-host disease (GvHD) despite the fact that the BiTE leads to a significant activation and expansion of donor-derived T-cells with a subsequent impressive graft-versus-leukemia (GvL) effect (4). Based on these observations, another cohort of 6 patients was treated with blinatumomab after they experienced multiple relapses after one or two allogeneic stem cell transplantations (5). Again, GvHD was not seen in these heavily pretreated patients. Another important observation was made in two patients in this cohort. These two patients had a high blast load prior to blinatumomab and did not respond to a first cycle and rather showed progressive disease. The progression could be halted by palliative chemotherapy and the patients then again received blinatumomab with a lower tumor load. Surprisingly, both patients experienced an MRD-negative CR. The side effects of the blinatumomab treatment in these patients were acceptable given their previous intensive anti-leukemic therapies including one or more allogeneic stem cell transplantations. Only two of the patients experienced a cytokine-release syndrome (CRS) grade III/IV. Based on these initial promising observations in the compassionate use of blinatumomab in pediatric patients <18 years of age with chemotherapy-refractory B-cell precursor ALL, an open-label phase I/II study was initiated and 49 patients were treated in phase I and 44 patients in phase II (6). The treatment consisted of multiple courses of blinatumomab given as continuous infusion over 4 and 2 weeks rest between the cycles. The primary endpoints were, to determine the maximum-tolerated dose (MTD) in the phase I part and to monitor the CR rate after two cycles in the phase II part. Four patients had a dose-limiting toxicity in the first cycle in the phase I part and the MTD was determined to be 15 µg/m2/day. Based on the data, the recommended dose was 5 µg/m2/day for 7 days followed by 15 µg/m2/day thereafter. Of the 70 patients who received the recommended dose, 27 (39%) achieved CR after the first two cycles and 14 (52%) of those patients had an MRD-negative CR. The most frequent adverse events ≥ grade 3 were anemia (36%), thrombocytopenia (21%) and hypokalemia (17%). Three patients and one patient had a grade 3 (4%) and grade 4 (1%) CRS, respectively.
These promising data led to the approval of blinatumomab for the treatment of pediatric patients with Philadelphia chromosome-negative (Ph−) refractory ALL. The restriction to Ph- ALL is based on the availability of alternative treatment strategies with tyrosine-kinase inhibitors (TKIs) for patients with Ph+ ALL, but not on the fact, that these patients do not respond to blinatumomab. In adult patients, blinatumomab showed the same efficacy in Ph+ ALL compared to Ph− ALL and 89% of patients with Ph+ ALL achieved a MRD-negative CR, irrespective of resistance to chemotherapy or therapy with TKIs (7).Various ongoing studies are under way, to evaluate the efficacy of blinatumomab in MRD-positive patients or to incorporate blinatumomab into current standard chemotherapeutic regimens [for review see (8)].
The emergence of CD19-negative ALL blasts has been observed in 10–20% of adult patients who relapsed after therapy with Blinatumomab (9,10). This immune escape seems to be rather due to a disrupted CD19 membrane trafficking than to the outgrowth of CD19-negative progenitor cells or myeloid lineage swift (11). However, a CD19-negative relapse with switch to a myeloid phenotype during therapy with Blinatumomab has been observed in a child with infant leukemia (12).
Attempts have been made to identify patients upfront, who will or will not respond to blinatumomab therapy. Recently, the response to blinatumomab was predicted via the level of regulatory T-cells (Tregs) at start of therapy (13). The Tregs were defined by the co-expression of CD4/CD25/FOXP3. Patients who responded to blinatumomab had a mean of 4.8% Tregs compared to 10.3% in non-responders. A cut-off of 8.5% was able to identify all responders to blinatumomab and excluded 70% of non-responders. In addition, a 1.7-fold upregulation of the programmed cell death protein 1 (PD-1) on the Tregs was observed in vitro after incubation of the cells with blinatumomab. In another study, a correlation between the expression of the PD-1 ligand (PD-L1) on the ALL blasts and the resistance to blinatumomab treatment was hypothesized (14). The expression of PD-L1 was increased in patients, refractory to blinatumomab treatment and exhaustion markers (PD-1, TIM-3) were significantly higher on patients’ T cells compared to physiologic controls. In addition, in vivo treatment of a patient with blinatumomab resulted in the induction of PD-L1 expression on the initially PD-L1 negative blasts. Based on these observations, a 12-year old patient, who relapsed after two allogeneic stem cell transplantations and who was refractory to blinatumomab and in whom the in vivo upregulation of PD-L1 expression during blinatumomab therapy was observed was treated subsequently with a combination of blinatumomab and the anti-PD1 checkpoint inhibitor pembrolizumab (14). The treatment with this combination was without acute toxicities and GvHD was not seen. However, she experienced an inflammatory response with high fever and increase of inflammatory parameters. A bone marrow aspiration at day 34 after start of the combined therapy showed a morphological CR. An increase of PD-L1 expression was also observed in an adult patient with B-precursor ALL resistant to blinatumomab (15). Consequently, a clinical trial is currently conducted in adult patients to evaluate the safety and efficacy in patients with refractory ALL (ClinicalTrials.gov Identifier: NCT03160079). Hopefully, similar studies will also be initiated in the near future in pediatric patients with ALL. Since long-term outcome data in pediatric patients are not yet available, it is currently advisable, that patients proceed to allogeneic stem cell transplantation, if they achieve a MRD-negative response after blinatumomab therapy and if they are clinically eligible for a transplant.
Chimeric antigen receptor (CAR) T-cells
CAR T cells are engineered T cells which are transduced with a CAR that couple single-chain Fv domains of antibodies against surface expressed tumor targets to the T cell receptor and thereby redirecting T cells to tumor cells positive for the corresponding target. Various modifications of the T cell activation signals have been introduced and according to the costimulatory domain, first, second and third generation CARs have been engineered [for review see (16)]. Imai et al. first reported the generation of a novel receptor (anti-CD19-BB-ζ) containing the signaling domain of 4-1BB (CD137) and showed a much higher cytotoxicity of these CAR T cells compared to cells lacking the 4-1BB (17). Based on this construct, researchers at the Children’s Hospital Philadelphia, USA, engineered anti-CD19 CAR T cells and treated two patients with recurrent and refractory ALL (18). Both treated patient achieved MRD-negative remission. One of the patients later relapsed with CD19-negative blasts. In a subsequent phase I study, 30 children with ALL were treated and CR was achieved in 27 (90%) patients (19). The 6-month event-free and overall survival was 67% and 78%, respectively. Expanding CD19 CAR T cells were seen in the 27 responding patients.
In the subsequent phase 2 multicenter study using CD19 CAR T cells (now named tisagenlecleucel) in children and young adults with ALL, 75 patients received an infusion of tisagenlecleucel (20). The overall remission rate within 3 months was 81% and all patients, who responded, had a MRD-negative CR. The rates of event-free and overall survival were 73% and 90%, respectively, at 6 months. Persistence of tisagenlecleucel was observed as long as 20 months. Grade 3 and 4 adverse events occurred in 73% of patients. Neurologic events were observed in 40%, which could be managed by supportive care. A major side effect was CRS, which occurred in 73% of the patients caused by a systemic inflammatory response with T-cell activation and proliferation. CRS is increasingly seen in patients receiving CAR T cells. A CRS grading system and suggestion for the clinical management of CRS has been established (21). Another phase I escalating study was performed in 21 patients with CD19 CAR T cells. The MTD in this study was defined as 1×106/kg CD19 CAR T cells. In 4 of the 21 patients, severe grade 4 CRS occurred. The CR rate was 70% with 12 of 20 patients achieving MRD-negative response (22). An additional phase I study evaluated the outcome of 45 patients, who received CD19 CAR T cells of a defined CD4/CD8 formulation (23). The overall intent-to-treat analysis of MRD-negative remission rate was 93% and 100% in patients who received a lymphodepletion using fludarabine and cyclophosphamide. Twenty-three percent of patients also experienced reversible severe CRS.
Antigen loss has been reported to be a cause of resistance to CD19 targeted therapies (24,25). Therefore, CD22-targeted CAR T cells have been engineered and given to 21 patients (26). Seventeen patients had received prior CD19-directed therapies, among them 15 who received CD19-targeted CAR T cells. In 10 patients, the lymphoblasts were negative for CD19 or expressed CD19 weakly (CD19dim), including 9 after CD19 CAR T cell therapy and 1 after treatment with blinatumomab. CRS occurred in 16 of the 21 patients. A robust expansion and persistence of the cells was seen and 12 patients (57%) achieved CR, and 9 of these patients had a MRD-negative CR. Another approach to prevent antigen escape might be the use of bispecific CARs and CD20/CD19 CARs (27) or CD19/CD22 CARs (28), where T cells express two different CARs (dual CAR) or where T cells express two single chains linked in line using the same signaling domain (tandem CARs) (29) . Clinical studies are currently performed in pediatric and young adult patients using CD19/CD22 dual CARS (ClinicalTrials.gov Identifier: NCT03330691) and a clinical study is currently ongoing at the Biotherapeutic Department and Hematology Department at the General Hospital Beijing, China (ClinicalTrials.gov Identifier: NCT03398967). In this phase I/II study, the safety allogenic gene-edited dual specificity CD19 and CD20 or CD22 CAR-T in patients with relapsed or refractory hematological malignancies is evaluated.
In summary, the development of CAR T cell therapy for CD19+ and/or CD22+ ALL has transformed the treatment of relapsed and refractory disease. However, severe toxicity including neurologic adverse events and mainly CRS have to be acknowledged and new approaches to prevent or ameliorate these adverse events will allow in the future to move this effective strategy into upfront treatment strategies, which might hopefully allow to reduce intensive chemotherapy and first promising results have been reported (30). In this case-controlled study in adult patients, CD19-targeted CAR T cell therapy was compared with reinduction chemotherapy and patients receiving CAR therapy had higher rates of remissions and longer survival. Similar studies are warranted in children, thus hopefully reducing heavy chemotherapy exposure and preventing long-term sequelae from chemotherapy. Whether the responses are long lasting in children and whether the patients still should proceed to allogeneic stem cell transplantation after achieving a MRD-negative remission is still not clear and each patient should be discussed on an individual basis.
Alloreactive natural killer (NK) cells
Attempts to exploit autologous NK cells in patients showed limited clinical efficacy, the focus of research was directed toward the use of NK cells from healthy related or unrelated donors in the context of allogeneic cell therapy [for review see (31)]. NK cell function is regulated by an array of receptors transducing either inhibitory or activatory signals (32). Among these receptors, killer immunoglobulin-like receptors (KIRs) are of great importance. KIRs recognize HLA A, B, and C alleles as their ligands. Alloreactivity is defined as the lack of inhibition, when donor NK cells express an inhibitory KIR, for which the respective ligand is absent on the recipient’s leukemic blasts (33). A strong GvL effect was mediated by alloreactive NK cells in adult patients with acute myeloid leukemia (AML) (34) and in children with ALL undergoing T-cell depleted HLA-haploidentical stem cell transplantation (35,36). Activating KIRs have also been identified and two KIR haplotypes can be distinguished: KIR haplotype A, which has a fixed number of genes encoding inhibitory receptors and KIR haplotype B, which has variable gene content and 1 or more activatory genes. Among individuals with haplotype B, a KIR B-content score can be established based on the number of mainly activatory genes. A reduced risk for relapse was seen in patients with AML given an allogeneic stem cell transplantation from unrelated KIR haplotype B donors (37). While this effect was not seen in adult patients with ALL in the unrelated HSCT setting, KIR B haplotype donors conferred a reduced risk for relapse after haploidentical transplantation in children with ALL (38). Moreover, a high KIR B-content score was associated with a significantly reduced risk for relapse. Therefore, careful donor selection plays an important role in allogeneic transplantation of children with ALL. An approach to overcome the inhibition of NK cells, mediated by the engagement of inhibitory receptors with their ligands, is the activation of NK cells via their FCGR3A receptor CD16. This activation overrides the inhibition and leads to augmentation of the NK activity (39). Based on these findings, an Fc-optimized anti-CD19 antibody was constructed and successfully used post-transplant in selected high risk patients with ALL (40).
AML
Analogue to blinatumomab, a CD3×CD33 BiTE construct named AMG330 was engineered to target AML (41). This BiTE is very effective in recruiting and activating autologous T cells in preclinical studies (42). Similar to the observation in ALL, the blockade of the PD-1/PD-L1 axis augmented the lysis of AML cells by this BiTE, which might unblocked lead to an immune escape of AML blasts (43). No clinical early phase studies have yet been reported in pediatric patients with refractory AML.
While the depletion of CD33+ blasts and CD33+ normal hematopoietic progenitor with the AMG330 BiTE would only be transient, CAR T cells might persist and lead to a permanent depletion of hematopoietic precursors with subsequent bone marrow aplasia. Currently, no AML-specific antigens have been identified, that might be safely used as targets for the CAR strategy. There are many attempts and numerous strategies to find safe approaches, including gene-editing techniques, antibodies and combination therapies [for review see (44)]. A promising target could be CLL-1, which is expressed on leukemia stem cells, but not in hematopoietic stem cells (HSCs). In a preclinical xenograft model of AML, CAR T cells directed against CLL-1 exerted a strong antileukemic activity, while normal HSCs were not targeted due to the lack of CLL-1 expression (45). Early phase clinical trials using CAR T cells for the treatment of refractory AML in children would be warranted.
Solid tumors
Neuroblastoma
Anti-GD2 antibodies
The disialoganglioside GD2 is highly expressed on almost all neuroblastoma cells and is therefore a suitable target for immunotherapeutic approaches and antibodies against GD2 have been clinically evaluated since the 1980s for the treatment of patients with high-risk neuroblastoma [for review see (46)]. Initially, murine anti-GD2 antibodies m3F8 (47) and murine m14.18 (48) showed promising anti-neuroblastoma activity in vitro and were further developed for clinical applications. The anti-neuroblastoma activity of both antibodies is mediated by human complement-dependent cytotoxicity (CDC) and the antibody-dependent cellular cytotoxicity (ADCC) mediated by Fc-receptor expressing effector cells including NK cells, monocytes and neutrophils. A phase I study with m3F8 showed promising clinical responses in 4 out of 8 patients (49). In six evaluable patients treated with the murine 14.G2a antibody derived from m14.18, a CR was observed in two patients (50). One of these patients had multiple relapses after autologous and allogeneic stem cell transplantation from an HLA-identical sibling and a CR was induced with the 14.G2a antibody. As of February 2018, this patient is still in remission >27 years after receiving the 14.G2a antibody (Handgretinger, unpublished observation). Common side effects of the m3F8 and 14.G2a were pain, hypertensions, fever, urticarial reactions and the induction of human anti-mouse antibodies (HAMAs), which resulted in the inactivation of the antibodies. In order to further exploit and enhance the ADCC activity, clinical studies combining the m3F8 with the granulocyte-macrophage colony-stimulating factor (GM-CSF) were performed and impressive responses were observed (51,52). In order to augment the ADCC of the 14.G2a antibody, a phase I/IB trial of 14.G2a in combination with Interleukin 2 (IL2) was conducted in 33 patients with refractory neuroblastoma (53). Seven of the 33 patients additionally received GM-CSF. In order to avoid the induction of HAMAs by the murine antibodies, the mouse/human chimeric antibody ch14.18 was developed. This antibody has the variable regions of the 14.G2a and the constant regions of a human IgG1 antibody (54). This chimeric antibody also mediates CDC, but has 50–100-fold higher ADCC activity compared to the 14.G2a antibody. In a first phase I study, 9 patients were treated with 19 courses of ch14.18 at various dose levels. A complete and partial remission was seen in 2 patients each. In contrast to the previous study using the murine 14.G2a antibody, none of the patients developed a HAMA response (55). Based on these initial data, 164 patients, who were treated according to the German neuroblastoma protocol NB97, received at least one cycle of ch14.18 treatment as a consolidation after intensive chemotherapy. The overall survival rate was better after consolidation with ch14.18 compared to no consolidation (56). The Children’s Oncology Group (COG) in the US initiated a phase I study of ch14.18 in combination with GM-CSF and IL-2 in patients after autologous bone marrow transplantation (57). Twenty-five patients were enrolled and the MTD was determined to be 25 mg/m2/day for 4 days. It was demonstrated that the addition of IL-2 and GM-CSF was tolerable in the early post-transplant period. Based on these findings, a larger study was performed, which compared in a randomized manner, the treatment with ch14.18, IL-2, GM-CSF and isotretinoin with isotretinoin alone (58). A total of 266 patients were randomized. An interim analysis showed a superiority of the antibody arm to the standard arm with regard to event-free survival (66%±5% vs. 46%±5% at 2 years, P=0.01) and overall survival (86%±4% vs. 75%±5% at 2 years, P=0.02). These results led to the approval of the ch14.18 (then named dinutuximab) by the US Food and Drug Administration (FDA) under the name of UnituxinTM (59). While the American dinutuximab was produced in SP2/0 murine myeloma cells, efforts were made in the SIOPEN study group to evaluate the efficacy of ch14.18 produced in mammalian Chinese hamster ovary (CHO) cells. In a phase I study, 16 patients were treated with ch14.18/CHO (then named dinutuximab-beta) as an 8-hour infusion for 5 consecutive days (60). The dose level of 20 mg/m2/day was confirmed and the side effects were very similar to ch14.18 produced in SP2/0 cells. The results of a phase III study regarding toxicity and outcome in the context of the HR-NBL1/SIOPEN trial were positive (61) and dinutuximab-beta recently received approval by the European Medical Agency (EMA) and is now available under the name of Dinutuximab-beta EUSA.
A major side effect of both ch14.18 antibodies is the induction of neuropathic pain, which may partially be attributed to the complement activation. Therefore, a humanized version of the ch14.18 has been engineered to diminish complement activation by a point mutation in the Fc region of the antibody (hu14.18K332A). In a rat model of allodynia, hu14.18K332A elicited less allodynia than ch14.18 due to a reduced complement activation (62). In a preclinical model, the antibody was still active against neuroblastoma despite the decreased CDC (63). In a phase I study, 38 patients received a median of 2 cycles of hu14.18K332A (64). The MTD and recommended dose for phase II studies was 60 mg/m2/day for 4 days. In a retrospective analysis, hu14.18K332A induced less pain than ch14.18 based on the opioid requirements (65). Further studies are currently evaluating hu14.18K332A in combination with IL-2, GM-CSF and haploidentical NK cells (66) or in combination with chemotherapy and NK cells (67). In parallel, a humanized version of the m3F8 was engineered to enhance ADCC and diminish CDC (68) and initial phase I trials with the hu3F8 showed that the adverse events in monotherapy were similar to m3F8 (69) and that the combination of hu3F8 and GM-CSF is feasible and safe (70). Another strategy to diminish pain is the reduction of the infusion rate. While the ch14.18 is normally infused over an 8-hour period, a 24-hour long-term infusion of 10 mg/m2/day over 10 days has been investigated in 53 patients in combination with IL-2 (71,72).
Immunocytokines (ICs)
Another way to concomitantly use antibodies and cytokines, is the use of ICs. These are monoclonal antibodies with a cytokine linked to the Fc part of the antibody [for review see (73)]. Higher concentrations of the cytokines at the tumor environment should induce an effective immune response, while minimizing the systemic side effects of the cytokine. In preclinical studies, the hu14.18-IL-2 IC was highly effective (74). In early clinical studies, the toxicity profile of the ICs was similar to the ones reported in patients receiving ch14.18 (75) and responses restricted to patients with low burden osteo medullary disease were reported (76).
CAR T cells
CAR T cells have been generated targeting GD2 and first generation CAR T cells were given to patients (77). Interestingly, neuropathic pain was not elicited by the CAR T cells and promising anti-neuroblastoma activity was seen. The CAR T cells persisted up to 192 weeks after injection (77). In a phase I study, patients were treated with a third-generation GD2-CAR. In order to enhance the CAR expansion, lymphodepleting chemotherapy consisting of fludarabine, cyclophosphamide and a PD-1 immune checkpoint inhibitor were concomitantly given (78). In the cohort treated only with CAR T cells, the cells were detectable whereas in the cohort receiving CAR T cells and lymphodepletion, an increase of CAR T expansion by up to 3 logs was observed. Additional PD-1 inhibition in a third cohort did not further enhance expansion or persistence of the CAR T cells. The antitumor response at week 6 was modest, which might be explained by the immunosuppressive tumor microenvironment and, which might prevent the infiltration of the tumor by the CAR T cells. A strategy to improve tumor infiltration and anti-tumor efficacy was evaluated in a preclinical orthotopic xenograft model of neuroblastoma. GD2-CAR T cells were given together with the inhibitor of angiogenesis bevacizumab, which led to a tumor vasculature remodelling and a massive infiltration of the tumors by the CAR T cells (79). The infiltrating CAR T cells produced large amounts of interferon-γ (IFN-γ), which resulted in the upregulation of PD-L1 on tumor cells and PD-1 on the T cells. Therefore, checkpoint inhibitors might further enhance the antitumor effect and clinical studies using this approach are warranted. However, in another human neuroblastoma xenograft model, high affinity GD2-CAR T cells induced fatal encephalitis with CAR T cell infiltration of the brain and neural destruction (80). Therefore, studies using GD2 CAR T cells should be conducted cautiously and should be well-monitored for such adverse events.
NK cells
NK cells and IL-2 activated NK cells can target and lyse neuroblastoma cells either spontaneously or more effectively via ADCC [for review see (81)]. It has been shown that the KIR mediated inhibition by certain HLA antigens can be overcome by activation of the NK cells via their Fc receptor with ch14.18 (82). In addition, the genotypes of KIR receptors, their HLA ligands and of the Fc receptors influenced the response to immunotherapy with the hu14.18-IL-2 IC (83) and KIR3DL1 allelic polymorphisms and HLA-B epitopes modulated the in vivo response to 3F8 and patients with lack of inhibition had a survival advantage (84). Patients’ KIR and KIR-ligand genotypes influenced also the outcome of patients receiving dinutuximab (85). Patients with Fc-gamma-receptor polymorphism 2A and 3A (FCGR2A and -3A) and stimulatory KIR2DS2 genotypes showed a higher ADCC and had an improved survival after treatment with ch14.18/CHO (86). The concomitant use of haploidentical NK cells selected for non-inhibition based on their KIR-HLA interaction is tested in phase I trials with 3F8 (87) and with hu14.18 (66). The safety of in vitro amplified HLA-haploidentical donor immune cell infusions was demonstrated in patients with neuroblastoma (88). It is noteworthy that none of the patients experienced acute nor chronic GvHD. Another approach exploiting haploidentical NK cells is the combination of myeloablative conditioning regimen followed by the transplantation of CD3/CD19-depleted peripheral mobilized stem cell grafts enriched with NK cells and the post-transplant treatment with ch14.18/CHO (ClinicalTrials.gov Identifier: NCT01919866). This study has reached the accrual goal of 60 patients and the preliminary results are promising (89). Similar to CAR T cells, NK CAR cells can also be engineered and be used in the treatment of tumors [for review see (90)]. The human NK leukemia cell line NK92 was engineered to express a GD2-specific CAR, which markedly enhanced the killing of neuroblastoma cells (91).
Other neuroblastoma target antigens
Other antigens highly expressed on neuroblastoma cells are B7-H3 (CD276) (92) and the recently reported expression of the GPC2 oncoprotein as a candidate for an immunotherapeutic target in neuroblastoma (93). Glypicans are a family of six (GPC1-6) glycosylphosphatidylinositol (GPI)-anchored, extracellular proteoglycan signaling co-receptors, which play different roles in growth factor signaling and cancer cell growth (94). In a preclinical model of disseminated neuroblastoma mouse model with metastases to clinical relevant sites such as spine, skull, legs and pelvis, CAR T cells targeting GPC2 were able to effectively eliminate neuroblastoma cells (95) and GPC2 might be a promising target for further immunotherapeutic approaches. The B7-H3 antigen is also a promising target for NK cells via their ADCC (96) or for CAR T-cells (97). Another promising antigen expressed on neuroblastoma is L1-CAM (CD171), which is recognized by the CE7 monoclonal antibody. In preclinical studies, CD171-directed CAR T cells were generated and the safety was demonstrated in rhesus macaques and the feasibility of generating CD171-directed CAR T cells was assessed in heavily pretreated patients with neuroblastoma (98).
In summary, neuroblastoma is the first pediatric solid tumor, for which immunotherapy has been shown to be effective. It took almost 30 years from the first applications of the murine anti-GD2 antibody until approval and anti-GD2 antibodies are now a component of multimodality therapy for patients with high-risk neuroblastoma. Early phase clinical studies using other immunotherapeutic approaches are being conducted or are in planning phases and it can be expected that further improvements will be made in the future, most likely by combinations of different immunotherapeutic strategies.
Brain tumors
The main pillars of therapy are maximal surgical resection, adjuvant radiotherapy and chemotherapy. Despite improvements in outcomes, survivors often suffer long-term toxicities of these treatments including neurological deficits and cognitive disorders, thus new therapies must be evaluated. A major barrier for better strategies is the blood brain barrier (BBB) and chemotherapeutic agents show limited penetration of the BBB. New immunotherapeutic approaches should overcome the BBB. The immune checkpoint inhibitor pembrolizumab is currently being evaluated in children with refractory/progressive high grade glioma, diffuse pontine gliomas (DIPGs) or hypermutated brain tumors (ClinicalTrials.gov Identifier: NCT02359565). Especially patients with hypermutant glioblastoma multiforme, caused by a biallelic germline mistmach repair deficiency, might benefit from the treatment with checkpoint inhibitors (99). Other strategies include cancer vaccines, oncolytic viral therapy and CAR T cells. Glioma associated peptide vaccines have been evaluated in HLA-A2—positive patients with HGGs and DIPGs (100). Thirteen of 21 children had an immune response to the targeted antigens. However, this approach is restricted to HLA-A2-positive patients. Another approach is the use of ex vivo activated autologous dendritic cells loaded with tumor antigen mRNA in patients with medulloblastoma (101) and a clinical study is currently being performed using this approach (ClinicalTrials.gov Identifier: NCT01326104). Oncolytic viral therapy is also currently being evaluated in pediatric phase I studies in patients with recurrent supratentorial tumors (ClinicalTrials.gov Identifier: NCT02457845) (102). CAR T cells have also been demonstrated to exert potent antitumor activity against adult glioblastomas by targeting human epidermal growth factor receptor 2 (HER2) or IL13Rα2 and a dramatic response has been reported in an adult patient with glioblastoma after intracavitary infusion of CAR T cells targeting IL13Rα2 (103). Other approaches comprise phase I studies of adoptive cell therapies in children with posterior fossa tumors by local injection of ex vivo expanded autologous NK cells via an ommaya reservoir (ClinicalTrials.gov Identifier: NCT02271711), or the use of allogeneic T or NK cells in the context of non-myeloablative haploidentical stem cell transplantation in phase II trials (ClinicalTrials.gov Identifier: NCT01804634 and ClinicalTrials.gov Identifier: NCT02100891). These trials are also open for patients with advanced sarcomas.
The BBB remains a significant barrier for effective treatment strategies, but activated T cells can cross this barrier and it is anticipated that CAR T cell based immunotherapeutic strategies will hopefully show efficacy and improve outcome of patients with recurrent/refractory brain tumors.
Sarcomas
While progress has been made in the treatment of localized sarcomas, the clinical outcome of disseminated soft tissue sarcomas, such as rhabdomyosarcoma, Ewing’s sarcoma, osteosarcoma and others is still dismal (104) and new therapeutic approaches are urgently needed. Immunotherapeutic approaches comprise antibodies against targets expressed on sarcoma cells, CAR T cells, immune checkpoint inhibitors and their combinations.
Monoclonal antibodies
Various antibodies have been and are explored in early phase clinical studies including antibodies directed against insulin growth factor 1 receptor (IGF-1R) (105) or directed against the HER2 (106) with only modest efficacies and no apparent survival benefit. A promising target antigen broadly expressed on almost all pediatric sarcomas is B7-H3 (CD276) (107). The function of B7-H3 is not fully understood, but recent studies support its role in inhibiting T-cell activation, proliferation and cytokine secretion indicating that B7-H3 might function as a immune checkpoint (108). Unfortunately, the receptor of the B7-H3 ligand has not yet been clearly identified, which would lead to a better understanding of the function of this interaction. A humanized Fc-optimized antibody against B7-H3 has been generated and is tested in adult patients with CD276+ neoplasms (109). This MGA271 antibody, also named enoblituzumab, is currently being tested in pediatric patients with solid tumors (ClinicalTrials.gov Identifier: NCT02982941). Another anti-CD276 antibody is the humanized 8H9 antibody, which has in vitro potent antitumor activity (110). This antibody has been used for intrathecal radioimmunotherapy in metastatic CNS neuroblastoma (111) and the radiolabeled 8H9 is currently evaluated in early phase studies in the treatment of refractory, recurrent, or advanced CNS or leptomeningeal cancer (ClinicalTrials.gov Identifier: NCT00089245) and in patients with desmoplastic small round cell tumors and other solid tumors involving the peritoneum (ClinicalTrials.gov Identifier: NCT0109964). Other anti-CD276 chimeric Fc-optimized antibodies and fusion proteins are currently being developed preclinically (own unpublished data).
ICs
ICs might be able to overcome the immunosuppressive effect of the tumor microenvironment by the targeted delivery of proinflammatory cytokines, to locally stimulate immune effector cells and anti-CD276-IL-2 or –IL15 ICs are currently being developed and have so far shown impressive antitumor activity in vitro (own unpublished data). A promising IC is NHS-IL-12 (112). Interleukin 12 (IL-12) is a pleiotropic proinflammatory cytokine produced by activated dendritic cells and promotes the differentiation of Th1 T cells (113). It also increases the proliferation and lytic activity of T and NK cells (114). The NHS antibody is directed against necrosis-associated antigens, which are abundantly expressed in tumors, but to a much lesser extent on normal tissue. The necrosis-targeted IL-12 immunocytokine NHS-IL12 was engineered by genetically fusion of two human IL-12 heterodimers to the C-termini of the heavy chains of the NHS76 antibody, which was selected for its specific ability to bind to necrotic regions of tumors in vivo (115). In a humanized mouse model of human rhabdomyosarcoma, it was demonstrated that NHS-IL-12 induced senescence and myogenic differentiation, most likely induced by the local induction of IFN-γ and tumor necrosis factor-α (116). In order to increase the local concentration of IL-12, low dose local tumor irradiation increased the uptake of radiolabeled NHS-IL-12 up to 8-fold in a xenograft model of rhabdomyosarcoma (117) and NHS-12 in combination with local irradiation induced an abscopal effect in vivo in a humanized rhabdomyosarcoma mouse model (118). A clinical study in adult patients with solid tumors and NHS-IL12 alone is currently recruiting (ClinicalTrials.gov Identifier: NCT01417546) and another study is evaluating the safety, tolerability and pharmacokinetics of the checkpoint inhibitor avelumab in combination with NHS-IL12 (ClinicalTrials.gov Identifier: NCT02994953). Similar studies are warranted for pediatric disseminated sarcomas. In a phase IB trial, the IC NHS-IL-2 was combined with radiotherapy in patients with metastatic non-small cell lung cancer following first line chemotherapy in adult patients. This combination was well-tolerated and 2 of 13 patients achieved long-term survival (119). Together with the preclinical data of Eckert et al. (118) in the humanized mouse model of rhabdomyosarcoma, combinations of NHS-12 or NHS-IL2 together with local low-dose irradiation and/or with checkpoint inhibitors are warranted for pediatric patients with sarcomas.
CARs and TRUCKs
While CAR T cells have shown exciting results in leukemia, there are numerous challenges in solid tumors, namely (I) to overcome the microenvironment and to increase the migration and infiltration of CAR T cells into solid tumors, bone metastases or the core of solid tumors (120), (II) to overcome the immunosuppressive microenvironment encountered in solid tumors (121,122) (III) to avoid on-target-off tumor toxicity, since most of the tumor-associated antigens are not tumor specific and might also be expressed on normal cells (123) and (IV) to avoid tumor escape mechanism by antigen loss (124). While many preclinical studies for the generation of anti-sarcoma CAR T cells are currently underway, only few clinical studies have been reported in pediatric sarcomas. A phase I/II study was conducted in patients with refractory HER2—positive sarcomas using CAR T cells expressing an HER2 specific receptor (125). Nineteen patients were enrolled at various dose levels. At doses of 1×105/m2 or higher, HER2-positive CAR T cells were detected 3 hours after infusion in 14 of 16 patients. The cells persisted for at least 6 weeks in 7 of 9 evaluable patients who received more than 1×106/m2 CAR T cells. Of 17 evaluable patients, 4 had stable disease for 12 weeks up to 14 months. In a resected tumor of one patient, ≥90% necrosis was found. The median survival of all 19 infused patients was 10.3 months (range, 5.1 to 29.1 months). Most importantly, no significant toxicity was seen, while a severe fatal adverse event was reported in an adult patient who received a total of 1×1010 HER2/neu targeting CAR T cells (126). Therefore, careful dose-escalating studies in pediatric sarcoma patients using other target antigens, such as CD276 or others are warranted.
An interesting approach to overcome the immunosuppressive effect of the tumor microenvironment is the use of TRUCKs (127). TRUCK stands for T Cell Redirected Cytokine Killing and describes the use of CAR T cells as a vehicle to produce and release large amounts of cytokines that accumulate in the solid tumor microenvironment and induce an inflammatory immune response. This strategy is similar to the described NHS-IL2 or –IL12 ICs, but might be more specific due to the CAR receptor. The feasibility of the TRUCK strategy was demonstrated by the local accumulation of IL-12 and T cells redirected by a tumor-targeting CAR and additionally transduced with a CAR-inducible IL-12 cassette, with secretion of IL-12 upon CAR recognition of the tumor antigen (128). More recently, CAR T cells, releasing the proinflammatory cytokine IL-18, have been engineered and augmented cytotoxic activity against advanced solid tumors has been demonstrated (129,130). Future clinical trials should show whether these cells hold their preclinical promises in the treatment of solid tumors (131).
Checkpoint inhibitors
Few studies have interrogated the efficacy of immune checkpoint inhibitors in pediatric tumors. While impressive responses have been observed in adults with solid tumors, less promising results have been seen in children. In a phase I clinical trial, the CTLA4 checkpoint inhibitor ipilimumab was evaluated in 33 patients with recurrent of progressive solid tumors (132). The toxicity profile was very similar to the one seen in adult patients, but no objective tumor response was observed. A clinical study evaluating the combination of nivolumab (PD-1 blocking antibody) and ipilimumab is ongoing (ClinicalTrials.gov Identifier: NCT02304458), but so far no objective response was seen in the nivolumab alone arm (133). A study evaluating pembrolizumab (anti-PD-1 antibody) showed a response rate of 6.1% (134) and in a study with atezolizumab (PD-L1 antibody), partial responses were seen in some patients with Hodgkin lymphoma. The induction of a tumor-specific T cell response is driven by the mutational landscape and the neo-antigen load of the tumors (135) and adult tumors with microsatellite instability have a very high mutational load and have been proven very sensitive to checkpoint inhibitors (136). Pediatric patients harboring homozygous germline mutations of mismatch repair genes have also a high mutational load (137) and this subgroup of patients might benefit from checkpoint inhibitors (99).
Conclusions
The benefit of immunotherapeutic strategies has been demonstrated in pediatric leukemia with blinatumomab, CD19-CAR T cells and to some extent with anti-GD2 antibodies in neuroblastoma. Many other immunotherapeutic strategies are currently being developed and evaluated in early phase clinical studies. A list of actively recruiting immunotherapeutic clinical trials is depicted in Table 1. Compared to the “soluble” hematologic malignancies, solid tumors are a major challenge for immunotherapy due to the microenvironment of solid tumors, which protects tumor cells from immunotherapeutic attacks. New strategies will be necessary to overcome the immune suppressive effects of the tumor microenvironment (Figure 1) and rational combination of different strategies will be necessary in the future to cure pediatric patients, who cannot be cured currently with standard treatments. Much more research and close interaction between researchers and clinicians will hopefully bring us to our final aim, namely to cure every child with cancer.
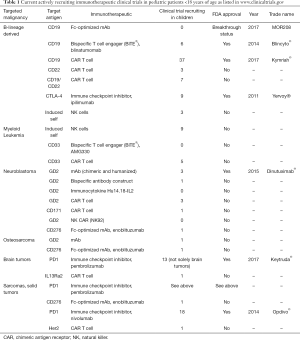
Full table
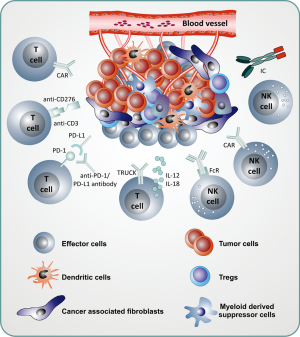
Acknowledgements
This work was supported from the “Stiftung für krebskranke Kinder Tübingen e.V.”, the Jose-Carreras Leukemia foundation and the “Stefan-Morsch-Stiftung”. We thank Peter Weber for the graphic art.
Footnote
Conflicts of Interest: The authors have no conflicts of interest to declare.
References
- Mack M, Riethmüller G, Kufer P. A small bispecific antibody construct expressed as a functional single-chain molecule with high tumor cell cytotoxicity. Proc Natl Acad Sci U S A 1995;92:7021-5. [Crossref] [PubMed]
- Mølhøj M, Crommer S, Brischwein K, et al. CD19-/CD3-bispecific antibody of the BiTE class is far superior to tandem diabody with respect to redirected tumor cell lysis. Mol Immunol 2007;44:1935-43. [Crossref] [PubMed]
- Bargou R, Leo E, Zugmaier G, et al. Tumor regression in cancer patients by very low doses of a T cell-engaging antibody. Science 2008;321:974-7. [Crossref] [PubMed]
- Handgretinger R, Zugmaier G, Henze G, et al. Complete remission after blinatumomab-induced donor T-cell activation in three pediatric patients with post-transplant relapsed acute lymphoblastic leukemia. Leukemia 2011;25:181-4. [Crossref] [PubMed]
- Schlegel P, Lang P, Zugmaier G, et al. Pediatric posttransplant relapsed/refractory B-precursor acute lymphoblastic leukemia shows durable remission by therapy with the T-cell engaging bispecific antibody blinatumomab. Haematologica 2014;99:1212-9. [Crossref] [PubMed]
- von Stackelberg A, Locatelli F, Zugmaier G, et al. Phase I/Phase II Study of Blinatumomab in Pediatric Patients With Relapsed/Refractory Acute Lymphoblastic Leukemia. J Clin Oncol 2016;34:4381-9. [Crossref] [PubMed]
- Martinelli G, Dombret H, Chevallier P, et al. Complete molecular and hematologic response in adult patients with relapsed/refractory (R/R) Philadelphia chromosome-positive B-precursor acute lymphoblastic leulemia (ALL) following treatment with Blinatumomab:Results from a phase 2 single-arm, multicenter study (ALCANTARA). Blood 2015;126:abstr 679.
- Queudeville M, Handgretinger R, Ebinger M. Immunotargeting relapsed or refractory precursor B-cell acute lymphoblastic leukemia - role of blinatumomab. Onco Targets Ther 2017;10:3567-78. [Crossref] [PubMed]
- Topp MS, Gökbuget N, Zugmaier G, et al. Long-term follow-up of hematologic relapse-free survival in a phase 2 study of blinatumomab in patients with MRD in B-lineage ALL. Blood 2012;120:5185-7. [Crossref] [PubMed]
- Topp MS, Gökbuget N, Zugmaier G, et al. Phase II trial of the anti-CD19 bispecific T cell-engager blinatumomab shows hematologic and molecular remissions in patients with relapsed or refractory B-precursor acute lymphoblastic leukemia. J Clin Oncol 2014;32:4134-40. [Crossref] [PubMed]
- Braig F, Brandt A, Goebeler M, et al. Resistance to anti-CD19/CD3 BiTE in acute lymphoblastic leukemia may be mediated by disrupted CD19 membrane trafficking. Blood 2017;129:100-4. [Crossref] [PubMed]
- Rayes A, McMasters RL, O'Brien MM. Lineage Switch in MLL-Rearranged Infant Leukemia Following CD19-Directed Therapy. Pediatr Blood Cancer 2016;63:1113-5. [Crossref] [PubMed]
- Duell J, Dittrich M, Bedke T, et al. Frequency of regulatory T cells determines the outcome of the T-cell-engaging antibody blinatumomab in patients with B-precursor ALL. Leukemia 2017;31:2181-90. [Crossref] [PubMed]
- Feucht J, Kayser S, Gorodezki D, et al. T-cell responses against CD19+ pediatric acute lymphoblastic leukemia mediated by bispecific T-cell engager (BiTE) are regulated contrarily by PD-L1 and CD80/CD86 on leukemic blasts. Oncotarget 2016;7:76902-19. [Crossref] [PubMed]
- Köhnke T, Krupka C, Tischer J, et al. Increase of PD-L1 expressing B-precursor ALL cells in a patient resistant to the CD19/CD3-bispecific T cell engager antibody blinatumomab. J Hematol Oncol 2015;8:111. [Crossref] [PubMed]
- Schubert ML, Hoffmann JM, Dreger P, et al. Chimeric antigen receptor transduced T cells: Tuning up for the next generation. Int J Cancer 2018;142:1738-47. [Crossref] [PubMed]
- Imai C, Mihara K, Andreansky M, et al. Chimeric receptors with 4-1BB signaling capacity provoke potent cytotoxicity against acute lymphoblastic leukemia. Leukemia 2004;18:676-84. [Crossref] [PubMed]
- Grupp SA, Kalos M, Barrett D, et al. Chimeric antigen receptor-modified T cells for acute lymphoid leukemia. N Engl J Med 2013;368:1509-18. [Crossref] [PubMed]
- Maude SL, Frey N, Shaw PA, et al. Chimeric antigen receptor T cells for sustained remissions in leukemia. N Engl J Med 2014;371:1507-17. [Crossref] [PubMed]
- Maude SL, Laetsch TW, Buechner J, et al. Tisagenlecleucel in Children and Young Adults with B-Cell Lymphoblastic Leukemia. N Engl J Med 2018;378:439-48. [Crossref] [PubMed]
- Lee DW, Gardner R, Porter DL, et al. Current concepts in the diagnosis and management of cytokine release syndrome. Blood 2014;124:188-95. Erratum in: Blood 2015;126:1048. [Crossref] [PubMed]
- Lee DW, Kochenderfer JN, Stetler-Stevenson M, et al. T cells expressing CD19 chimeric antigen receptors for acute lymphoblastic leukaemia in children and young adults: a phase 1 dose-escalation trial. Lancet 2015;385:517-28. [Crossref] [PubMed]
- Gardner RA, Finney O, Annesley C, et al. Intent-to-treat leukemia remission by CD19 CAR T cells of defined formulation and dose in children and young adults. Blood 2017;129:3322-31. [PubMed]
- Sotillo E, Barrett DM, Black KL, et al. Convergence of Acquired Mutations and Alternative Splicing of CD19 Enables Resistance to CART-19 Immunotherapy. Cancer Discov 2015;5:1282-95. [Crossref] [PubMed]
- Gardner R, Wu D, Cherian S, et al. Acquisition of a CD19-negative myeloid phenotype allows immune escape of MLL-rearranged B-ALL from CD19 CAR-T-cell therapy. Blood 2016;127:2406-10. [Crossref] [PubMed]
- Fry TJ, Shah NN, Orentas RJ, et al. CD22-targeted CAR T cells induce remission in B-ALL that is naive or resistant to CD19-targeted CAR immunotherapy. Nat Med 2018;24:20-8. [Crossref] [PubMed]
- Martyniszyn A, Krahl AC, André MC, et al. CD20-CD19 Bispecific CAR T Cells for the Treatment of B-Cell Malignancies. Hum Gene Ther 2017;28:1147-57. [Crossref] [PubMed]
- Qin H, Haso W, Nguyen S, et al. Preclinical development of bispecific chimeric antigen receptor targeting both CD19 and CD22. Blood 2015;126:abstr 4427.
- Schneider D, Xiong Y, Wu D, et al. A tandem CD19/CD20 CAR lentiviral vector drives on-target and off-target antigen modulation in leukemia cell lines. J Immunother Cancer 2017;5:42. [Crossref] [PubMed]
- Wei G, Hu Y, Pu C, et al. CD19 targeted CAR-T therapy versus chemotherapy in re-induction treatment of refractory/relapsed acute lymphoblastic leukemia: results of a case-controlled study. Ann Hematol 2018;97:781-9. [PubMed]
- Handgretinger R, Lang P, André MC. Exploitation of natural killer cells for the treatment of acute leukemia. Blood 2016;127:3341-9. [Crossref] [PubMed]
- Moretta L, Locatelli F, Pende D, et al. Killer Ig-like receptor-mediated control of natural killer cell alloreactivity in haploidentical hematopoietic stem cell transplantation. Blood 2011;117:764-71. [Crossref] [PubMed]
- Moretta A, Bottino C, Pende D, et al. Identification of four subsets of human CD3-CD16+ natural killer (NK) cells by the expression of clonally distributed functional surface molecules: correlation between subset assignment of NK clones and ability to mediate specific alloantigen recognition. J Exp Med 1990;172:1589-98. [Crossref] [PubMed]
- Ruggeri L, Capanni M, Urbani E, et al. Effectiveness of donor natural killer cell alloreactivity in mismatched hematopoietic transplants. Science 2002;295:2097-100. [Crossref] [PubMed]
- Leung W, Iyengar R, Turner V, et al. Determinants of antileukemia effects of allogeneic NK cells. J Immunol 2004;172:644-50. [Crossref] [PubMed]
- Pfeiffer MM, Feuchtinger T, Teltschik HM, et al. Reconstitution of natural killer cell receptors influences natural killer activity and relapse rate after haploidentical transplantation of T- and B-cell depleted grafts in children. Haematologica 2010;95:1381-8. [Crossref] [PubMed]
- Cooley S, Weisdorf DJ, Guethlein LA, et al. Donor selection for natural killer cell receptor genes leads to superior survival after unrelated transplantation for acute myelogenous leukemia. Blood 2010;116:2411-9. [Crossref] [PubMed]
- Oevermann L, Michaelis SU, Mezger M, et al. KIR B haplotype donors confer a reduced risk for relapse after haploidentical transplantation in children with ALL. Blood 2014;124:2744-7. [Crossref] [PubMed]
- Lang P, Barbin K, Feuchtinger T, et al. Chimeric CD19 antibody mediates cytotoxic activity against leukemic blasts with effector cells from pediatric patients who received T-cell-depleted allografts. Blood 2004;103:3982-5. [Crossref] [PubMed]
- Seidel UJ, Schlegel P, Grosse-Hovest L, et al. Reduction of Minimal Residual Disease in Pediatric B-lineage Acute Lymphoblastic Leukemia by an Fc-optimized CD19 Antibody. Mol Ther 2016;24:1634-43. [Crossref] [PubMed]
- Aigner M, Feulner J, Schaffer S, et al. T lymphocytes can be effectively recruited for ex vivo and in vivo lysis of AML blasts by a novel CD33/CD3-bispecific BiTE antibody construct. Leukemia 2013;27:1107-15. [Crossref] [PubMed]
- Laszlo GS, Gudgeon CJ, Harrington KH, et al. Cellular determinants for preclinical activity of a novel CD33/CD3 bispecific T-cell engager (BiTE) antibody, AMG 330, against human AML. Blood 2014;123:554-61. [Crossref] [PubMed]
- Krupka C, Kufer P, Kischel R, et al. Blockade of the PD-1/PD-L1 axis augments lysis of AML cells by the CD33/CD3 BiTE antibody construct AMG 330: reversing a T-cell-induced immune escape mechanism. Leukemia 2016;30:484-91. [Crossref] [PubMed]
- Fan M, Li M, Gao L, et al. Chimeric antigen receptors for adoptive T cell therapy in acute myeloid leukemia. J Hematol Oncol 2017;10:151. [PubMed]
- Wang J, Chen S, Xiao W, et al. CAR-T cells targeting CLL-1 as an approach to treat acute myeloid leukemia. J Hematol Oncol 2018;11:7. [Crossref] [PubMed]
- Sait S, Modak S. Anti-GD2 immunotherapy for neuroblastoma. Expert Rev Anticancer Ther 2017;17:889-904. [Crossref] [PubMed]
- Kushner BH, Cheung NK. Clinically effective monoclonal antibody 3F8 mediates nonoxidative lysis of human neuroectodermal tumor cells by polymorphonuclear leukocytes. Cancer Res 1991;51:4865-70. [PubMed]
- Mujoo K, Cheresh DA, Yang HM, et al. Disialoganglioside GD2 on human neuroblastoma cells: target antigen for monoclonal antibody-mediated cytolysis and suppression of tumor growth. Cancer Res 1987;47:1098-104. [PubMed]
- Cheung NK, Lazarus H, Miraldi FD, et al. Ganglioside GD2 specific monoclonal antibody 3F8: a phase I study in patients with neuroblastoma and malignant melanoma. J Clin Oncol 1987;5:1430-40. [Crossref] [PubMed]
- Handgretinger R, Baader P, Dopfer R, et al. A phase I study of neuroblastoma with the anti-ganglioside GD2 antibody 14.G2a. Cancer Immunol Immunother 1992;35:199-204. [Crossref] [PubMed]
- Kushner BH, Kramer K, Cheung NK. Phase II trial of the anti-G(D2) monoclonal antibody 3F8 and granulocyte-macrophage colony-stimulating factor for neuroblastoma. J Clin Oncol 2001;19:4189-94. [Crossref] [PubMed]
- Cheung NK, Cheung IY, Kramer K, et al. Key role for myeloid cells: phase II results of anti-G(D2) antibody 3F8 plus granulocyte-macrophage colony-stimulating factor for chemoresistant osteomedullary neuroblastoma. Int J Cancer 2014;135:2199-205. [Crossref] [PubMed]
- Frost JD, Hank JA, Reaman GH, et al. A phase I/IB trial of murine monoclonal anti-GD2 antibody 14.G2a plus interleukin-2 in children with refractory neuroblastoma: a report of the Children's Cancer Group. Cancer 1997;80:317-33. [Crossref] [PubMed]
- Mueller BM, Romerdahl CA, Gillies SD, et al. Enhancement of antibody-dependent cytotoxicity with a chimeric anti-GD2 antibody. J Immunol 1990;144:1382-6. [PubMed]
- Handgretinger R, Anderson K, Lang P, et al. A phase I study of human/mouse chimeric antiganglioside GD2 antibody ch14.18 in patients with neuroblastoma. Eur J Cancer 1995;31A:261-7. [Crossref] [PubMed]
- Simon T, Hero B, Faldum A, et al. Long term outcome of high-risk neuroblastoma patients after immunotherapy with antibody ch14.18 or oral metronomic chemotherapy. BMC Cancer 2011;11:21. [Crossref] [PubMed]
- Gilman AL, Ozkaynak MF, Matthay KK, et al. Phase I study of ch14.18 with granulocyte-macrophage colony-stimulating factor and interleukin-2 in children with neuroblastoma after autologous bone marrow transplantation or stem-cell rescue: a report from the Children's Oncology Group. J Clin Oncol 2009;27:85-91. [Crossref] [PubMed]
- Yu AL, Gilman AL, Ozkaynak MF, et al. Anti-GD2 antibody with GM-CSF, interleukin-2, and isotretinoin for neuroblastoma. N Engl J Med 2010;363:1324-34. [Crossref] [PubMed]
- Dhillon S. Dinutuximab: first global approval. Drugs 2015;75:923-7. Erratum in: Drugs 2015;75:1831. [Crossref] [PubMed]
- Ladenstein R, Weixler S, Baykan B, et al. Ch14.18 antibody produced in CHO cells in relapsed or refractory Stage 4 neuroblastoma patients: a SIOPEN Phase 1 study. MAbs 2013;5:801-9. [Crossref] [PubMed]
- Ladenstein R, Poetschger U, Gray J, et al. Toxicity and outcome of anti-GD2 antibody ch14.18/CHO in front-line, high-risk patients with neuroblastoma: Final results of the phase III immunotherapy randomisation (HR-NBL1/SIOPEN trial). J Clin Oncol 2016;34:10500.
- Sorkin LS, Otto M, Baldwin WM 3rd, et al. Anti-GD(2) with an FC point mutation reduces complement fixation and decreases antibody-induced allodynia. Pain 2010;149:135-42. [Crossref] [PubMed]
- Otto M, Barfield RC, Martin WJ, et al. Combination immunotherapy with clinical-scale enriched human gammadelta T cells, hu14.18 antibody, and the immunocytokine Fc-IL7 in disseminated neuroblastoma. Clin Cancer Res 2005;11:8486-91. [Crossref] [PubMed]
- Navid F, Sondel PM, Barfield R, et al. Phase I trial of a novel anti-GD2 monoclonal antibody, Hu14.18K322A, designed to decrease toxicity in children with refractory or recurrent neuroblastoma. J Clin Oncol 2014;32:1445-52. [Crossref] [PubMed]
- Anghelescu DL, Goldberg JL, Faughnan LG, et al. Comparison of pain outcomes between two anti-GD2 antibodies in patients with neuroblastoma. Pediatr Blood Cancer 2015;62:224-8. [Crossref] [PubMed]
- Talleur AC, Triplett BM, Federico S, et al. Consolidation Therapy for Newly Diagnosed Pediatric Patients with High-Risk Neuroblastoma Using Busulfan/Melphalan, Autologous Hematopoietic Cell Transplantation, Anti-GD2 Antibody, Granulocyte-Macrophage Colony-Stimulating Factor, Interleukin-2, and Haploidentical Natural Killer Cells. Biol Blood Marrow Transplant 2017;23:1910-7. [Crossref] [PubMed]
- Federico SM, McCarville MB, Shulkin BL, et al. A Pilot Trial of Humanized Anti-GD2 Monoclonal Antibody (hu14.18K322A) with Chemotherapy and Natural Killer Cells in Children with Recurrent/Refractory Neuroblastoma. Clin Cancer Res 2017;23:6441-9. [Crossref] [PubMed]
- Cheung NK, Guo H, Hu J, et al. Humanizing murine IgG3 anti-GD2 antibody m3F8 substantially improves antibody-dependent cell-mediated cytotoxicity while retaining targeting in vivo. Oncoimmunology 2012;1:477-86. [Crossref] [PubMed]
- Basu EM, Kushner B, Modak S, et al. Phase I study of anti-GD2 humanized 3F8 (hu3F8) monoclonal antibody (MAb) in patients with relapsed or refractory neuroblastoma (NB) or other GD2-positive solid tumors. Advances in Neuroblastoma Research 2014. Cologne 2014:POC034.
- Cheung IY, Kushner BH, Modak S, et al. Phase I trial of anti-GD2 monoclonal antibody hu3F8 plus GM-CSF: Impact of body weight, immunogenicity and anti-GD2 response on pharmacokinetics and survival. Oncoimmunology 2017;6:e1358331. [Crossref] [PubMed]
- Ceylan K, Jahns LJ, Lode BN, et al. Inflammatory response and treatment tolerance of long-term infusion of the anti-GD(2) antibody ch14.18/CHO in combination with interleukin-2 in patients with high-risk neuroblastoma. Pediatr Blood Cancer 2018;65:e26967. [Crossref] [PubMed]
- Mueller I, Ehlert K, Endres S, et al. Tolerability, response and outcome of high-risk neuroblastoma patients treated with long-term infusion of anti-GD(2) antibody ch14.18/CHO. MAbs 2018;10:55-61. [Crossref] [PubMed]
- Neri D, Sondel PM. Immunocytokines for cancer treatment: past, present and future. Curr Opin Immunol 2016;40:96-102. [Crossref] [PubMed]
- Hank JA, Surfus JE, Gan J, et al. Activation of human effector cells by a tumor reactive recombinant anti-ganglioside GD2 interleukin-2 fusion protein (ch14.18-IL2). Clin Cancer Res 1996;2:1951-9. [PubMed]
- Osenga KL, Hank JA, Albertini MR, et al. A phase I clinical trial of the hu14.18-IL2 (EMD 273063) as a treatment for children with refractory or recurrent neuroblastoma and melanoma: a study of the Children's Oncology Group. Clin Cancer Res 2006;12:1750-9. [Crossref] [PubMed]
- Shusterman S, London WB, Gillies SD, et al. Antitumor activity of hu14.18-IL2 in patients with relapsed/refractory neuroblastoma: a Children's Oncology Group (COG) phase II study. J Clin Oncol 2010;28:4969-75. [Crossref] [PubMed]
- Louis CU, Savoldo B, Dotti G, et al. Antitumor activity and long-term fate of chimeric antigen receptor-positive T cells in patients with neuroblastoma. Blood 2011;118:6050-6. [Crossref] [PubMed]
- Heczey A, Louis CU, Savoldo B, et al. CAR T Cells Administered in Combination with Lymphodepletion and PD-1 Inhibition to Patients with Neuroblastoma. Mol Ther 2017;25:2214-24. [Crossref] [PubMed]
- Bocca P, Di Carlo E, Caruana I, et al. Bevacizumab-mediated tumor vasculature remodelling improves tumor infiltration and antitumor efficacy of GD2-CAR T cells in a human neuroblastoma preclinical model. Oncoimmunology 2017;7:e1378843. [Crossref] [PubMed]
- Richman SA, Nunez-Cruz S, Moghimi B, et al. High-Affinity GD2-Specific CAR T Cells Induce Fatal Encephalitis in a Preclinical Neuroblastoma Model. Cancer Immunol Res 2018;6:36-46. [Crossref] [PubMed]
- Wang W, Erbe AK, Hank JA, et al. NK Cell-Mediated Antibody-Dependent Cellular Cytotoxicity in Cancer Immunotherapy. Front Immunol 2015;6:368. [Crossref] [PubMed]
- Tarek N, Le Luduec JB, Gallagher MM, et al. Unlicensed NK cells target neuroblastoma following anti-GD2 antibody treatment. J Clin Invest 2012;122:3260-70. [Crossref] [PubMed]
- Delgado DC, Hank JA, Kolesar J, et al. Genotypes of NK cell KIR receptors, their ligands, and Fcγ receptors in the response of neuroblastoma patients to Hu14.18-IL2 immunotherapy. Cancer Res 2010;70:9554-61. [Crossref] [PubMed]
- Forlenza CJ, Boudreau JE, Zheng J, et al. KIR3DL1 Allelic Polymorphism and HLA-B Epitopes Modulate Response to Anti-GD2 Monoclonal Antibody in Patients With Neuroblastoma. J Clin Oncol 2016;34:2443-51. [Crossref] [PubMed]
- Erbe AK, Wang W, Carmichael L, et al. Neuroblastoma Patients' KIR and KIR-Ligand Genotypes Influence Clinical Outcome for Dinutuximab-based Immunotherapy: A Report from the Children's Oncology Group. Clin Cancer Res 2018;24:189-96. [Crossref] [PubMed]
- Siebert N, Jensen C, Troschke-Meurer S, et al. Neuroblastoma patients with high-affinity FCGR2A, -3A and stimulatory KIR 2DS2 treated by long-term infusion of anti-GD(2) antibody ch14.18/CHO show higher ADCC levels and improved event-free survival. Oncoimmunology 2016;5:e1235108. [Crossref] [PubMed]
- Modak S, Cheung N, Kushner BH, et al. Phase I study of haploidentical Natural Killer (NK) cells plus monoclonal antibody 3F8 for resistant high-risk neuroblastoma (HR-NB). Proc.Advances in Neurblastoma Research (ANR) 2014;130:abstr 075.
- Zhang F, Sun XF, Li YQ, et al. Safety of in vitro amplified HLA-haploidentical donor immune cell infusions for childhood malignancies. Chin J Cancer 2013;32:661-6. [Crossref] [PubMed]
- Lang P, Illhardt T, Ebinger M, et al. Haploidentical stem cell transplantation and subsequent immunotherapy with anti-GD2 antibody for patients with relapsed metastatic neuroblastoma. J Clin Oncol 2015;33:abstr 10056.
- Hu Y, Tian ZG, Zhang C. Chimeric antigen receptor (CAR)-transduced natural killer cells in tumor immunotherapy. Acta Pharmacol Sin 2018;39:167-76. [Crossref] [PubMed]
- Esser R, Müller T, Stefes D, et al. NK cells engineered to express a GD2 -specific antigen receptor display built-in ADCC-like activity against tumour cells of neuroectodermal origin. J Cell Mol Med 2012;16:569-81. [Crossref] [PubMed]
- Castriconi R, Dondero A, Augugliaro R, et al. Identification of 4Ig-B7-H3 as a neuroblastoma-associated molecule that exerts a protective role from an NK cell-mediated lysis. Proc Natl Acad Sci U S A 2004;101:12640-5. [Crossref] [PubMed]
- Bosse KR, Raman P, Zhu Z, et al. Identification of GPC2 as an Oncoprotein and Candidate Immunotherapeutic Target in High-Risk Neuroblastoma. Cancer Cell 2017;32:295-309.e12. [Crossref] [PubMed]
- Matas-Rico E. Glycerophosphodiesterase GDE2 Promotes Neuroblastoma Differentiation through Glypican Release and Is a Marker of Clinical Outcome. Cancer Cell 2016;30:548-62. [Crossref] [PubMed]
- Li N, Fu H, Hewitt SM, et al. Therapeutically targeting glypican-2 via single-domain antibody-based chimeric antigen receptors and immunotoxins in neuroblastoma. Proc Natl Acad Sci U S A 2017;114:E6623-31. [Crossref] [PubMed]
- Bottino C, Dondero A, Bellora F, et al. Natural killer cells and neuroblastoma: tumor recognition, escape mechanisms, and possible novel immunotherapeutic approaches. Front Immunol 2014;5:56. [Crossref] [PubMed]
- Picarda E, Ohaegbulam KC, Zang X. Molecular Pathways: Targeting B7-H3 (CD276) for Human Cancer Immunotherapy. Clin Cancer Res 2016;22:3425-31. [Crossref] [PubMed]
- Künkele A, Taraseviciute A, Finn LS, et al. Preclinical Assessment of CD171-Directed CAR T-cell Adoptive Therapy for Childhood Neuroblastoma: CE7 Epitope Target Safety and Product Manufacturing Feasibility. Clin Cancer Res 2017;23:466-77. [Crossref] [PubMed]
- Bouffet E, Larouche V, Campbell BB, et al. Immune Checkpoint Inhibition for Hypermutant Glioblastoma Multiforme Resulting From Germline Biallelic Mismatch Repair Deficiency. J Clin Oncol 2016;34:2206-11. [Crossref] [PubMed]
- Pollack IF, Jakacki RI, Butterfield LH, et al. Antigen-specific immune responses and clinical outcome after vaccination with glioma-associated antigen peptides and polyinosinic-polycytidylic acid stabilized by lysine and carboxymethylcellulose in children with newly diagnosed malignant brainstem and nonbrainstem gliomas. J Clin Oncol 2014;32:2050-8. [Crossref] [PubMed]
- Nair SK, Driscoll T, Boczkowski D, et al. Ex vivo generation of dendritic cells from cryopreserved, post-induction chemotherapy, mobilized leukapheresis from pediatric patients with medulloblastoma. J Neurooncol 2015;125:65-74. [Crossref] [PubMed]
- Saha D, Martuza RL, Rabkin SD. Macrophage Polarization Contributes to Glioblastoma Eradication by Combination Immunovirotherapy and Immune Checkpoint Blockade. Cancer Cell 2017;32:253-67.e5. [Crossref] [PubMed]
- Brown CE, Alizadeh D, Starr R, et al. Regression of Glioblastoma after Chimeric Antigen Receptor T-Cell Therapy. N Engl J Med 2016;375:2561-9. [Crossref] [PubMed]
- Perkins SM, Shinohara ET, DeWees T, et al. Outcome for children with metastatic solid tumors over the last four decades. PLoS One 2014;9:e100396. [Crossref] [PubMed]
- Weigel B, Malempati S, Reid JM, et al. Phase 2 trial of cixutumumab in children, adolescents, and young adults with refractory solid tumors: a report from the Children's Oncology Group. Pediatr Blood Cancer 2014;61:452-6. [Crossref] [PubMed]
- Ebb D, Meyers P, Grier H, et al. Phase II trial of trastuzumab in combination with cytotoxic chemotherapy for treatment of metastatic osteosarcoma with human epidermal growth factor receptor 2 overexpression: a report from the children's oncology group. J Clin Oncol 2012;30:2545-51. [Crossref] [PubMed]
- Modak S, Kramer K, Gultekin SH, et al. Monoclonal antibody 8H9 targets a novel cell surface antigen expressed by a wide spectrum of human solid tumors. Cancer Res 2001;61:4048-54. [PubMed]
- Pardoll DM. The blockade of immune checkpoints in cancer immunotherapy. Nat Rev Cancer 2012;12:252-64. [Crossref] [PubMed]
- Powderly J, Core G, Flaherty K, et al. Interim results of an ongoing PhaseI, dose escalation study of MGA271 (Fc-optimized humanized anti-B7-H3 monoclonal antibody) in patients with refractory B7-H3-expressing neoplasms or neoplasms whose vasculature expresses B7-H3. J Immunother Cancer 2015;3:O8. [Crossref]
- Ahmed M, Cheng M, Zhao Q, et al. Humanized Affinity-matured Monoclonal Antibody 8H9 Has Potent Antitumor Activity and Binds to FG Loop of Tumor Antigen B7-H3. J Biol Chem 2015;290:30018-29. [Crossref] [PubMed]
- Kramer K, Kushner BH, Modak S, et al. Compartmental intrathecal radioimmunotherapy: results for treatment for metastatic CNS neuroblastoma. J Neurooncol 2010;97:409-18. [Crossref] [PubMed]
- Fallon J, Tighe R, Kradjian G, et al. The immunocytokine NHS-IL12 as a potential cancer therapeutic. Oncotarget 2014;5:1869-84. [Crossref] [PubMed]
- Manetti R, Parronchi P, Giudizi MG, et al. Natural killer cell stimulatory factor (interleukin 12 [IL-12]) induces T helper type 1 (Th1)-specific immune responses and inhibits the development of IL-4-producing Th cells. J Exp Med 1993;177:1199-204. [Crossref] [PubMed]
- Perussia B, Chan SH, D'Andrea A, et al. Natural killer (NK) cell stimulatory factor or IL-12 has differential effects on the proliferation of TCR-alpha beta+, TCR-gamma delta+ T lymphocytes, and NK cells. J Immunol 1992;149:3495-502. [PubMed]
- Sharifi J, Khawli LA, Hu P, et al. Characterization of a phage display-derived human monoclonal antibody (NHS76) counterpart to chimeric TNT-1 directed against necrotic regions of solid tumors. Hybrid Hybridomics 2001;20:305-12. [Crossref] [PubMed]
- Schilbach K, Alkhaled M, Welker C, et al. Cancer-targeted IL-12 controls human rhabdomyosarcoma by senescence induction and myogenic differentiation. Oncoimmunology 2015;4:e1014760. [Crossref] [PubMed]
- Eckert F, Schmitt J, Zips D, et al. Enhanced binding of necrosis-targeting immunocytokine NHS-IL12 after local tumour irradiation in murine xenograft models. Cancer Immunol Immunother 2016;65:1003-13. [Crossref] [PubMed]
- Eckert F, Jelas I, Oehme M, et al. Tumor-targeted IL-12 combined with local irradiation leads to systemic tumor control via abscopal effects in vivo. Oncoimmunology 2017;6:e1323161. [Crossref] [PubMed]
- van den Heuvel MM, Verheij M, Boshuizen R, et al. NHS-IL2 combined with radiotherapy: preclinical rationale and phase Ib trial results in metastatic non-small cell lung cancer following first-line chemotherapy. J Transl Med 2015;13:32. [Crossref] [PubMed]
- Long AH, Haso WM, Shern JF, et al. 4-1BB costimulation ameliorates T cell exhaustion induced by tonic signaling of chimeric antigen receptors. Nat Med 2015;21:581-90. [Crossref] [PubMed]
- Caruana I, Savoldo B, Hoyos V, et al. Heparanase promotes tumor infiltration and antitumor activity of CAR-redirected T lymphocytes. Nat Med 2015;21:524-9. [Crossref] [PubMed]
- Long AH, Highfill SL, Cui Y, et al. Reduction of MDSCs with All-trans Retinoic Acid Improves CAR Therapy Efficacy for Sarcomas. Cancer Immunol Res 2016;4:869-80. [Crossref] [PubMed]
- Li W, Song X, Jin Y, et al. CARTs for Solid Tumors: Feasible or Infeasible? Oncol Res Treat 2017;40:540-6. [Crossref] [PubMed]
- Hegde M, Mukherjee M, Grada Z, et al. Tandem CAR T cells targeting HER2 and IL13Rα2 mitigate tumor antigen escape. J Clin Invest 2016;126:3036-52. [Crossref] [PubMed]
- Ahmed N, Brawley VS, Hegde M, et al. Human Epidermal Growth Factor Receptor 2 (HER2) -Specific Chimeric Antigen Receptor-Modified T Cells for the Immunotherapy of HER2-Positive Sarcoma. J Clin Oncol 2015;33:1688-96. [Crossref] [PubMed]
- Morgan RA, Yang JC, Kitano M, et al. Case report of a serious adverse event following the administration of T cells transduced with a chimeric antigen receptor recognizing ERBB2. Mol Ther 2010;18:843-51. [Crossref] [PubMed]
- Chmielewski M, Hombach AA, Abken H. Of CARs and TRUCKs: chimeric antigen receptor (CAR) T cells engineered with an inducible cytokine to modulate the tumor stroma. Immunol Rev 2014;257:83-90. [Crossref] [PubMed]
- Chmielewski M, Hombach A, Heuser C, et al. T cell activation by antibody-like immunoreceptors: increase in affinity of the single-chain fragment domain above threshold does not increase T cell activation against antigen-positive target cells but decreases selectivity. J Immunol 2004;173:7647-53. [Crossref] [PubMed]
- Chmielewski M, Abken H. CAR T Cells Releasing IL-18 Convert to T-Bet(high) FoxO1(low) Effectors that Exhibit Augmented Activity against Advanced Solid Tumors. Cell Rep 2017;21:3205-19. [Crossref] [PubMed]
- Kunert A, Chmielewski M, Wijers R, et al. Intra-tumoral production of IL18, but not IL12, by TCR-engineered T cells is non-toxic and counteracts immune evasion of solid tumors. Oncoimmunology 2017;7:e1378842. [Crossref] [PubMed]
- Abken H. Driving CARs on the Highway to Solid Cancer: Some Considerations on the Adoptive Therapy with CAR T Cells. Hum Gene Ther 2017;28:1047-60. [Crossref] [PubMed]
- Merchant MS, Wright M, Baird K, et al. Phase I Clinical Trial of Ipilimumab in Pediatric Patients with Advanced Solid Tumors. Clin Cancer Res 2016;22:1364-70. [Crossref] [PubMed]
- Davis KL, Fox E, Reid JM, et al. ADVL1412: Initial results of a phase I/II study of nivolumab and ipilimumab in pediatric patients with relapsed/refractory solid tumorsGÇöA COG study. JCO 2017;35:10526.
- Geoerger B, Kang HJ, Yalon-Oren M, et al. Phase 1/2 KEYNOTE-051 study of pembrolizumab (pembro) in pediatric patients (pts) with advanced melanoma or a PD-L1+ advanced, relapsed, or refractory solid tumor or lymphoma. JCO 2017;35:10525.
- Majzner RG, Heitzeneder S, Mackall CL. Harnessing the Immunotherapy Revolution for the Treatment of Childhood Cancers. Cancer Cell 2017;31:476-85. [Crossref] [PubMed]
- Le DT, Uram JN, Wang H, et al. PD-1 Blockade in Tumors with Mismatch-Repair Deficiency. N Engl J Med 2015;372:2509-20. [Crossref] [PubMed]
- Shlien A, Campbell BB, de Borja R, et al. Combined hereditary and somatic mutations of replication error repair genes result in rapid onset of ultra-hypermutated cancers. Nat Genet 2015;47:257-62. [Crossref] [PubMed]