What is the role of immunotherapy in breast cancer?
Introduction
The immune system plays a complex role in the recognition/prevention, early eradication as well as progression of cancer. The hypothesis of “immunoediting” describes three distinct phases of host immunosurveillance and response; elimination, equilibrium and escape (1). In the elimination phase, the innate and adaptive immune system work to detect cancer and eradicate it. Putatively, rare cancer cells that survive the elimination phase enter an equilibrium phase where the adaptive immune system prevents further tumor outgrowth and maintain them in a state of dormancy without complete tumor eradication. In the escape phase, tumor cells acquire the ability to circumvent immune recognition and elimination through a broad variety of mechanisms (1). Hence, tumor evasion of the immune system is a hallmark of cancer (2).
Enhancement of innate immune system mechanisms to treat cancer was initiated several decades ago with high dose interleukin-2 demonstrating durable clinical benefit in patients with advanced malignancies, most notably melanoma and renal cell carcinoma (3,4). More recently, there has been significant resurgence in the use of immuno-oncology approaches following the identification of clinically relevant inhibitory pathways of T cell activation; the CTLA-4 and PD-1/PD-L1 axes (5,6). Blocking these pathways via immune checkpoint monoclonal antibody inhibitors has proven successful in a variety of cancers especially melanoma, renal cell carcinoma and non-small cell lung carcinoma. Importantly, following treatment with checkpoint inhibitors, durable clinical responses have been observed along with an increase in the proportion of patients surviving beyond the historical median as previously reported with conventional chemotherapeutics.
The molecular definition of breast cancer evolved from the initial identification of the estrogen receptor (ER) positive and negative subtypes followed by the human epidermal growth factor receptor 2 (HER2) positive or negative subtypes through to the more recent gene expression subtyping. Whilst these changes have led to refinement of stratified systemic therapy strategies, triple negative breast cancers (TNBC) and the luminal B ER-positive, HER2-negative breast cancers, still have limited treatment options and poorer prognosis as compared to their luminal A or HER2 enriched counterparts. The early clinical data of a small number of meaningful clinical responses to immunotherapy in breast cancer is leading towards a rational approach to therapeutic targeting of the immune system that can be adopted through understanding why the host immune system has failed in natural tumor suppression as well as the processes evolved by the tumor to circumvent an active immune system.
The immune response and cancer
Key to the initiation of an immune response against cancer is host immune system recognition of abnormally expressed tumor associated antigens (TAA). TAAs of suitable sizes and sequences are produced by partial digestion of parental tumor by cellular proteasome (7). Following combination with the major histocompatibility complex (MHC) within the endoplasmic reticulum, the TAA/MHC complex is presented at the cell surface (7,8). MHC class I (MHC-1) and MHC class II (MHC-2) both share this task of antigen presentation (8). MHC-1 complexes are presented on most nucleated cells whilst MHC-2 on antigen presenting cells (APC) such as dendritic cells (DC), macrophages or B cells. CD8-positive (CD8+) cytotoxic T lymphocytes (CTL) and CD4-positive (CD4+) T helper cells respectively can recognize these TAA/MHC complexes and activate a signaling cascade which ultimately triggers cellular destruction (8,9). The immune system consists of a tightly regulated system of checks and balances to protect the host from “self-destruction”. T cell activation is modulated both by stimulatory and inhibitory signals. Activation requires dual signaling both from the MHC peptides and from binding of co-stimulatory peptides such as CD28 which are present on T cell surfaces and specialized cells such as APCs (9). CD28 maintains T cell response through interaction with its ligands B7-1 (CD80) and B7-2 (CD86). CTL-associated antigen-4 (CTLA-4) is another cell surface molecule which binds with greater avidity to B7-1 and B7-2 ligands. Binding of CTLA-4 to its ligands shifts the balance towards an inhibitory signal resulting in T cell anergy instead of activation (10).
The programmed death 1 (PD1)-programmed death ligand 1 (PD-L1) pathway is another mechanism of regulating the immune system. Upon activation, CD4+ T cells, CD8+ T cells, natural killer (NK) T cell, B cells and monocytes express PD1 (6). PD1 binds to one of two ligands, PD-L1 and PD-L2 generating an inhibitory signal resulting in T cell anergy (8). PD-L1, is more broadly expressed on B cells, DC, macrophages and T cells in addition to non-hematopoietic cells and at sites of immune privilege such as the placenta (6). The role of these inhibitory pathways is crucial in tolerance to self-antigens, promoting fetal-maternal tolerance, protecting tissues from autoimmune attacks and controlling immune-mediated tissue damage during infections (6).
In cancer, although antigens are present, tumors may be “invisible” to the immune system due to the loss or modification of surface antigens and lack of expression of co-stimulatory molecules (11). In addition, tumors can escape elimination becoming clinical diseases through processes which include activating negative regulatory pathways, enhancement of an immune-inhibitory tumor microenvironment through infiltration of inhibitory immune cells [regulatory T cells (Tregs) and myeloid-derived suppressor cells (MDSC)] and elaboration of inhibitory cytokines [e.g., transforming growth factor-ß (TGFβ), interleukin-10 (IL-10) etc.] (11,12).
Rationale for immunotherapy in breast cancer
There are several lines of evidence in support of adopting an immune targeted approach in breast cancer.
Tumor infiltrating lymphocytes (TILs)
TILs are mononuclear immune cells which are observed in and around cancer cells. They consist of a combination of immune cell types including T lymphocytes, B lymphocytes, NK cells, macrophages and DC (13). The quantification of TILs is presently being used to imply tumor immunogenicity. Lymphocytic infiltration of breast cancer has been extensively reported (14,15). Consistent with older reports, assessment of TILs using contemporary standardized scoring methodology has identified TILs to be associated with aggressive tumor features such as high histological grade, hormone receptor negativity and high Ki67 expression (16). In the analysis of 2009 breast cancer samples from the BIG 02-98 phase 3 adjuvant study, TILs were higher in TNBC and HER2-positive cohorts as compared to the ER-positive HER2-negative cohort (16). There are robust and consistent evidence from prospective-retrospective adjuvant chemotherapy and chemotherapy-trastuzumab studies reporting positive associations of increased levels of TILs with survival end points in TNBC and HER2-positive breast cancer (16-19). Chemotherapy is thought to interact with the immune system, inducing “immunogenic” tumor cell death which can result in induction of antitumor immunity as well as immunomodulation by depletion of immunosuppressive Tregs or MDSC (20). It is postulated that chemotherapy contributes to improving immune response to TIL-rich breast cancers, thereby improving their elimination, reducing micro-metastases and resulting in more favorable outcomes.
Immunomodulatory genes
Several groups have identified distinct subgroups of breast cancer patients beyond standard clinicopathological features (21-23). In a large comprehensive meta-analysis integrating gene expression and clinicopathological data of over 2,100 breast cancer patients, the immune response gene expression signature was described to be significantly associated with clinical outcomes in the ER-negative, HER2-negative as well as the HER2-positive breast cancer subgroups (24). High expression levels of immune related genes were associated with better outcomes. Similar transcriptional studies of TNBC by separate groups have consistently identified distinct TNBC subtypes displaying unique ontologies and characterized by differing clinical prognoses and response to therapies (23,25,26). Two distinguishable subtypes of TNBC have been identified by the presence or absence of TILs and immune cell markers and signaling (23,26). The immune activated/responsive TNBCs are defined by the presence of TILs and interferon (IFN)/signal transducer and activator of transcription (STAT) signaling. This subtype of TNBC has the best prognosis, a lower risk of relapse; and opens up a tantalizing prospect of identifying patients who may benefit from immune checkpoint inhibitors (23,26). Subsequently, work by Doherty et al. demonstrated a link between repressed IFN/STAT expression signature and a mesenchymal cancer stem cell like phenotype which was reversed to a non-aggressive epithelial non stem cell like phenotype with IFN-ß treatment (27).
Programmed death ligand 1 (PD-L1) expression
The Cancer Genome Atlas (TCGA) RNA sequencing data demonstrated higher PD-L1 expression in TNBC versus non-TNBC samples (28). Immunohistochemical staining for PD-L1 expression as defined by more than 5% membranous staining using the 5H1 antibody was identified in approximately 19% of the 105 assessed TNBC tumors (28). In a separate study, of the 44 breast cancer specimens evaluated by immunostaining using the MIH1 clone PD-L1 antibody, 50% stained for the expression of PD-L1 in the tumor cells and/or TILs (29). The expression of PD-L1 was significantly associated with high histological grade (P=0.012), negative ER (P=0.036) and negative PR (P=0.04) (29).
Mutational burden
To drive an effective anti-tumor immune response, the host immunosurveillance must be able to identify TAA. Preclinical studies have identified neoantigens as products of somatic cancer mutations driving antitumor immune response (30). An increase in mutational load is therefore predicted to be associated with increased antigenicity and immunogenicity (31). Tumor types such as melanoma and lung cancer, which have high mutational burdens and predicted higher numbers of neoantigens, are more responsive to checkpoint immunotherapies (32,33). The molecular profile and landscape of somatic mutations in breast cancer has been well described (34,35). Amongst the breast cancer subtypes, a higher mutational load was observed in ER negative tumors (36). Germline mutations in BRCA1 and 2 genes account for about 5% to 10% of all breast cancers (37). BRCA mutated tumors are homologous repair (HR) deficient and dependent on the error-prone non-homologous end joining mechanism for DNA repair. The result is genomic instability and a predicted increase in mutational burden as compared to non BRCA mutated tumors. In a study characterizing the tumor neoantigen repertoire of 20 germline BRCA mutated breast cancers, the neoantigen load was found to vary significantly with a moderate load of 50–150 predicted neoantigens in half of the breast cancer patients studied, suggesting that a subset of these patients may benefit from an immune targeted strategy (38). HR deficiency is however complex and extends beyond germline mutations of the BRCA gene in a concept referred to as ‘BRCAness’. Much effort has been focused on identifying molecular features of tumors with characteristics similar to germline BRCA mutated tumors. Signatures of mutational processes imprinting a particular pattern of mutation has been described in breast cancer (35). A large study analyzing whole-genome sequences of 560 breast cancer cases identified rearrangement mutation signatures which were characterized by tandem duplications or deletions and associated with a defect in homologous-recombination-based DNA repair (35). More recently, a weighted model called HRDetect has been shown to accurately detect individuals with breast cancer harbouring BRCA1/BRCA2 mutations and identify additional tumors with germline and somatic mutations or in cases where BRCA mutations were previously not identified (39). Further validation of HRDetect in a separate cohort of breast cancers revealed up to 22% are BRCA deficient and potentially enriched for genomically unstable tumors (40).
Approach to targeting the immune system in breast cancer
There is a rapidly expanding compendium of strategies to overcome the diminution of immune stimulation through (I) revealing tumor antigens (vaccines) (II) elimination of cancer induced immunosuppression (checkpoint inhibitors) and (III) increasing the number of immune effector cells (adoptive T cell therapy).
Vaccines
Cancer vaccines are designed to prime the immune system to recognize TAAs and generate an immunological response specific to the cancer cells. Exploiting the adaptive immune system is appealing in its potential to result in immunological memory providing long term protection against the cancer without prolonged courses of therapy. Vaccines currently in development include peptide-based vaccines and cellular vaccines. Currently, no vaccine is approved by the U.S. Food and Drug Administration (FDA) for the treatment of breast cancer. On their own, vaccines are not effective in breast cancer patients with active metastatic cancers given the immunosuppressive nature of the metastatic tumor microenvironment and are probably more useful in those with minimal disease. This therapeutic strategy is an ongoing area of active research being evaluated for both primary and secondary prevention of breast cancer.
An example of a peptide-based vaccine, and the vaccine most advanced in development is the HER2 derived E75 peptide vaccine, nelipepimut-S (Neuvax, Galenda Biopharma). Nelipepimut-S was shown to have minimal toxicities and efficacious in raising E75-specific immunity in vaccinated HLA-A2+ and HLA-A3+ patients as measured by increasing CD8+ CTL and delayed type hypersensitivity reaction (41). Nelipepimut-S in combination with granulocyte-macrophage colony stimulating factor (GM-CSF) was evaluated as adjuvant therapy to prevent disease recurrence in patients with lymph node positive and high-risk lymph node negative patients in a combination of clinical trials (42). The 24-month combined analysis of these trials demonstrated disease recurrence in 5.6% of the vaccinated patients versus 13.1% of controls with a corresponding disease free survival (DFS) rate of 94.3% versus 86.8% (P=0.08) (42). Importantly, this trial identified a group of patients whose tumors have low HER2 expression who benefited from E75 vaccination with a much more robust immunologic response after vaccination than patients whose tumors overexpress HER2 (42). The DFS in patients with low HER2 expressing [defined by immunohistochemistry (IHC) 1+ or 2+] tumors was 94% in vaccinated patients versus 79.4% for controls (P=0.04) (42). Based on these encouraging results, the PRESENT trial (ClinicalTrials.gov identifier: NCT01479244), a phase 3 registration trial evaluating Nelipepimut-S in the prevention of recurrence in early stage node-positive breast cancers with low to intermediate HER2 expression was initiated but unfortunately terminated early due to futility following interim analysis on recommendations from the Independent Data Monitoring Committee (IDMC).
Cellular vaccines are vaccines which use either whole cells or cell lysates as a source of antigens presented ex vivo to patient derived mononuclear cells such as DC before being returned to patients (43). The group led by Dr. Brian Czerniecki developed a HER2 peptide pulsed DC vaccine engineered to polarize to the DC1 phenotype which induces a strong anti-HER2 immune response (44). DC1 have potent antigen presenting capacity sensitizing both effector T cells as well as CD4+ helper cells potentiating T cell response. In a study evaluating the safety, immune and clinical responses of the vaccine, Czerniecki et al. randomized 54 patients with biopsy proven HER2 positive ductal carcinoma in situ (DCIS) to vaccination via intralesional, intranodal or a combination of both six-weekly injections prior to surgery (45). The majority (81% of 53 patients evaluable for immune response) had a new or increased protocol specified immunogenic response following vaccination (45). The pathological complete response (pCR) rate in patients with pure DCIS without invasive cancer was 28.6% (45). Compared to historical responses of invasive cancers to DC vaccine, the achieved pCR rate is impressive and adds to the bulk of evidence suggesting vaccines as a strategy is more effective in patients with pre-invasive or early disease with low tumor burden.
Checkpoint inhibitors
Two classes of immune blockade inhibitors (checkpoint inhibitor) have now entered the clinic: CTLA-4 blockers and blockers of the PD-L1/PD1 inhibitory pathway. Stunning successes have been seen first in the field of melanoma, renal cell carcinoma and non-small cell lung cancer, indications for which checkpoint inhibitors are already standard of care.
CTLA4
Ipilimumab (Yervoy, Bristol-Myers Squibb) and tremelimumab (AstraZeneca) are monoclonal antibodies which bind to CTLA-4 preventing interaction with its ligands B7-1 and B7-2 (10). Ipilimumab is currently approved for use in the treatment of unresectable metastatic melanoma on the basis of improvement in overall survival (OS) with ipilimumab of 10 vs. 6 months with tumor vaccine [hazard ratio (HR) 0.66, 95% confidence interval (CI): 0.51–0.87] (46). In breast cancer, minimal activity has been seen thus far. Tremelimumab has been evaluated in combination with exemestane in a phase 1 study of 26 patients with previously treated advanced ER+ breast cancer (47). No responses were seen, 11 out of 26 (42%) patients had stable disease for more than 12 weeks. The group evaluated peripheral blood mononuclear cells (PBMC) by flow cytometry observing a marked increase in the ratio of biotinylated anti-human inducible costimulatory (ICOS)+ T cells to circulating FoxP3 Treg cells suggesting immune activation (47).
PD-1/PD-L1
The two PD-1 binding antibodies currently approved for clinical use in other indications are nivolumab (Opdivo, Bristol-Myers Squibb) and pembrolizumab (Keytruda, Merck). There are currently three PD-L1 targeted antibodies also approved for use in other indications: atezolizumab (Tecentriq, Genentech), durvalumab (Imfinzi, AstraZeneca UK Limited) and avelumab (Bavencio, EMD Serono). Given the multiple lines of evidence in support for targeting the PD1/PD-L1 pathway in TNBC, a major focus in development of PD1/PD-L1 inhibitors is in this group of patients. Table 1 summarizes notable studies of PD1/PD-L1 inhibitor monotherapy in the treatment of advanced breast cancer.
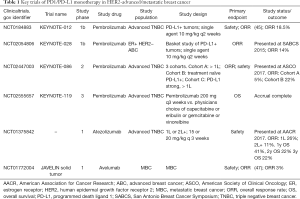
Full table
KEYNOTE-012 is a non-randomized multi-cohort phase 1b study evaluating the safety, tolerability and efficacy of pembrolizumab in patients with PD-L1 positive advanced TNBC, gastric cancer, urothelial cancer and head and neck cancer (48). Approximately 59% of patients with metastatic TNBC were PD-L1 expressing tumors as defined by PD-L1 expression in ≥1% of tumor or stroma using the prototype assay and 22C3 antibody. The study enrolled 32 heavily pre-treated TNBC patients. Overall 37.5% of patients experienced a decrease in tumor size which was durable. The overall response rate (ORR) was 18.5% with 1 complete response (CR) and 4 partial responses (PR). Three responders remained on study for over a year which is unprecedented given the aggressive natural history of this disease with a median OS of approximately 12 months (49). Of note, patients who had rapidly progressive disease identified through elevated LDH levels as a surrogate did not derive benefit from pembrolizumab which in a cohort of TNBC was associated with a long median time to response of 18 weeks (range, 7 to 32 weeks). On the basis of these results, KEYNOTE-086 (NCT02447003), a two-part multi-cohort non-randomized phase 2 study of pembrolizumab monotherapy in metastatic TNBC was initiated. Cohort A included patients who had previously received at least one line of treatment for metastatic disease regardless of PD-L1 status whilst cohort B included treatment naïve patients with PD-L1 positive tumors. Preliminary data from both cohorts were presented by Dr. Sylvia Adams at the American Society of Clinical Oncologist (ASCO) annual meeting in 2017. Of the 170 patients enrolled in cohort A, approximately 60% had PD-L1 positive tumors. The ORR was approximately 5%. Twenty seven percent of patients had a decrease in size of target lesions from baseline which was sustained. In contrast, the ORR of the 52 patients enrolled onto cohort B was significantly higher at 22% (Adams et al., ASCO 2017). Early trial data indicate that patients treated earlier in the course of metastatic disease are more likely to derive benefit from immune checkpoint inhibitors. We await results of KEYNOTE-119 (NCT02555657), a phase 3 study of pembrolizumab monotherapy versus single agent chemotherapy of physician’s choice in previously treated (1 or 2 prior lines of systemic therapy) metastatic TNBC which has now completed accrual.
Atezolizumab was evaluated as a single agent in an expansion cohort of metastatic TNBC patients in a phase 1a study (NCT01375842). Data from 115 evaluable TNBC patients was presented by Dr. Peter Schmid at the American Association for Cancer Research (AACR) annual meeting in July 2017. Two-thirds of the tumors had PD-L1 expression as defined by ≥5% of positive PD-L1 staining in immune-infiltrating cells. The ORR based on immune-related Response Evaluation Criteria in Solid Tumors (irRECIST) in 112 evaluable patients was 26% and 11% in the first line and second line and beyond setting respectively. Notably, the median duration of response was 21 months and median OS of responders was not reached. Higher response rates were seen in patients who were treated in the first line setting, had stronger PD-L1 staining and had tumors with more TILs and CD8 positive T cells (Schmid et al., AACR 2017). This study supports the rationale for the development of atezolizumab in earlier line treatment of metastatic TNBC.
In other subtypes of breast cancer, the role of PD1 inhibition in the ER-positive HER2-negative breast cancer was evaluated in KEYNOTE-028, an ongoing open label multi-cohort phase 1b basket study exploring the safety and efficacy of pembrolizumab in patients with PD-L1 expressing tumors defined by membranous PD-L1 expression in ≥1% of tumor, associated inflammatory cells or stroma using the prototype assay and 22C3 antibody. Data from the ER positive HER2 negative advanced breast cancer patient cohort was presented by Dr. Hope Rugo at the San Antonio Breast Cancer Symposium (SABCS) in 2015. Nineteen percent of the 248 patients screened were PD-L1 positive; 25 heavily pre-treated patients were included in the study. The ORR was 14%. The median time to response was eight weeks with all three responders showing fairly durable response remaining on study for more than 26 weeks (Rugo et al. SABCS 2015). Lastly, in the phase 1b JAVELIN solid tumor trial, avelumab was evaluated in 168 patients with pre-treated advanced breast cancer (50). Approximately 43% of patients had hormone receptor-positive, HER2-negative disease, 34% TNBC and 16% HER2 positive breast cancer. Patients had received a median of three prior therapies for metastatic disease. The confirmed ORR in the entire cohort was a disappointing 3% (95% CI: 1.0–6.8). There was a signal of greater activity amongst patients with TNBC. Out of the eight responders, five had TNBC (50).
Studies of monotherapy PD1/PD-L1 inhibitors are indicative of a signal of activity in breast cancer, however, single agent therapy has only benefitted a small proportion of patients. The minority, who respond, have a substantially longer duration of response than that ever seen with conventional chemotherapeutics. Efforts have now shifted to evaluating combinatory strategies where PD-1/PD-L1 inhibitors are used as a backbone in combination with therapies with complementary mechanisms of actions and non-overlapping toxicities (Figure 1). It is hypothesized that this strategy may further enhance the immune response broadening the group of patients who would benefit from an immune based cancer treatment.
Combinatory strategies
Chemo-immunotherapy combinations
Chemotherapy can be combined with immunotherapy to augment antitumor activity in a few ways. Firstly, chemotherapy can be used for cytoreduction and to achieve a state of minimal residual disease altering the balance of disease burden in favour of the T cell response. Secondly, chemotherapy can promote tumor antigen presentation and priming of tumor specific T cells. Lastly, chemotherapy can stimulate immune effectors either directly or through altering host immune-inhibition. We direct you to an excellent review article eloquently discussing chemotherapy and its effects on the immune system (51).
In the non-metastatic setting, pembrolizumab is combined with standard therapy of paclitaxel followed by doxorubicin and cyclophosphamide as neoadjuvant treatment for patients with locally advanced TNBC or ER-positive, HER2-negative breast cancers in the I-SPY2 trial. I-SPY2 is a novel adaptively randomized phase 2 trial which employs an algorithm to efficiently identify combinations of molecular subtypes of breast cancer and promising neoadjuvant regimens. Therapies that reach an 85% or greater predicted probability of success are moved forward to be tested in the I-SPY3 phase 3 program (52). Results of the pembrolizumab plus standard neoadjuvant therapy of weekly paclitaxel followed by doxorubicin plus cyclophosphamide were presented at ASCO 2017 (Nanda et al., ASCO 2017). Sixty-nine patients were randomized to four cycles of three-weekly pembrolizumab concurrent with paclitaxel. Compared to control, pembrolizumab tripled the estimated pCR rate in TNBC and is predicted to have a more than 99% chance of succeeding in a phase 3 trial. In the ER-positive, HER-2 negative arm, pembrolizumab increased the estimated pCR by 21% and is predicted to have an 88% chance of being successful in a phase 3 trial. There were slightly more grade 3 and above fatigue and nausea. Immune related toxicities were consistent with previous reports but of note, there were higher rates of adrenal insufficiency being reported in six patients, three of which was related to hypophysitis (Nanda et al., ASCO 2017).
Table 2 summarizes notable studies of PD1/PDL1 inhibitors in non-metastatic breast cancer. KEYNOTE-522 (NCT03036488) is an ongoing rapidly recruiting phase 3 randomized, double-blind study evaluating neoadjuvant pembrolizumab plus chemotherapy versus placebo plus chemotherapy followed by pembrolizumab versus placebo as adjuvant therapy for TNBC. Atezolizumab is similarly being developed in this setting. IMpassion-031 (NCT03197935) is a phase 3 double blind, randomized, placebo-controlled study evaluating the safety and efficacy of atezolizumab and nab-paclitaxel followed by doxorubicin and cyclophosphamide or placebo and the same regimen in stage 2 to 3 TNBC.
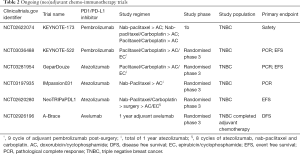
Full table
In the metastatic setting, nab-paclitaxel is one of the first chemotherapeutic agents to be established as an effective partner in combination with checkpoint inhibitor. Nab-paclitaxel is an albumin-bound paclitaxel which has greater tissue penetration and does not require steroid premedication. Nab-paclitaxel has been combined with atezolizumab in a phase 1b trial in metastatic TNBC reported at ASCO 2016 (Adams et al., ASCO 2016). The combination was fairly well tolerated with 53% all grade neutropenia and no dose limiting toxicities or treatment related deaths. ORR among 24 evaluable patients was approximately 71%, with higher responses seen in patients being treated in the first line. Responses were seen regardless of PD-L1 status. Three phase 3 trials (Table 3) evaluating atezolizumab with chemotherapy in earlier stage of metastatic disease are ongoing. IMpassion-130 (NCT02425891) and 131 (NCT03125902) evaluate the combination of atezolizumab with nab-paclitaxel and paclitaxel respectively as first line therapy for metastatic TNBC whilst IMpassion-132 (NCT03371017) evaluates atezolizumab in combination with investigators selected chemotherapy (gemcitabine/carboplatin or capecitabine) in early relapsing TNBC who have not received chemotherapy for advanced disease.
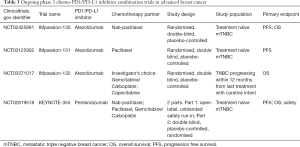
Full table
In the same disease setting, pembrolizumab in combination with chemotherapy is being evaluated in KEYNOTE 355, a two-part randomized double blind phase 3 study of pembrolizumab plus one of three chemotherapy regimens—nab-paclitaxel, paclitaxel or gemcitabine/carboplatin in previously untreated metastatic TNBC (NCT02819518) (Table 3). Eribulin, a microtubule inhibitor currently approved for the treatment of previously treated MBC has been combined with pembrolizumab in a phase 1b/2 study in patients with previously treated metastatic TNBC. Interim analysis of this phase 1b/2 study were first presented at SABCS in 2016 and updated in 2017. The recommended phase 2 dose was eribulin 1.4 mg/m2 on days 1 and 8 and pembrolizumab 200 mg on day 1 of a 21-day cycle. There were no dose limiting toxicities observed. The most frequent grade 3 and above adverse events were neutropenia and fatigue. The ORR was approximately 25% irrespective of PD-L1 status. A randomized phase 2 study of eribulin with or without pembrolizumab for ER-positive HER2-negative MBC has been initiated (NCT03051659).
Targeted therapy in combination with immune checkpoint inhibitors
HER2 overexpression is associated with high levels of proliferation, high histological grade and a higher level of TILs suggesting HER2-positive breast cancers could also be treated with immunotherapy approaches. In addition to direct cytotoxicity, HER2 directed therapy such as trastuzumab also elicit antibody dependent immune response. Nelipepimut-S is being evaluated as adjuvant therapy in combination with trastuzumab in a randomized phase 2 study of high risk HER2-positive breast cancer as defined by residual disease post neoadjuvant chemotherapy or lymph node positive disease in those who had initial surgery (NCT02297698). The PANACEA (IBCSG 45-13/BIG 4-13) trial (NCT0219556) is a single arm phase 1b/2 study evaluating pembrolizumab in combination with trastuzumab in trastuzumab-resistant HER2-positive MBC. Results were reported by Dr. Sherene Loi at SABCS 2017. Out of 146 patients screened, 68 were PD-L1-positive as defined initially by ≥1% tumor or stroma staining by the QualTek PD-L1 IHC assay and subsequently changed to combined positive score (CPS) of ≥1% using the 22C3 antibody. A total of 58 patients were enrolled. The ORR was 15%, disease control rate (DCR) of 24% and no responses were observed in the PD-L1-negative cohort. Consistent with prior reports with checkpoint inhibitors, responding patients achieved durable disease control of a median of 11 months (Loi et al., SABCS 2017). Trastuzumab-emtansine (TDM-1), the HER2-targeted antibody-drug conjugate is currently being evaluated in combination with pembrolizumab (NCT03032107) and atezolizumab (NCT02924883) in a phase 1 and 2 trial, respectively.
About 20% of breast cancers have mutation signatures of HR deficiency. Agents promoting DNA damage such as poly (ADP-ribose) polymerase (PARP)inhibitors results in anti-tumor response in BRCA mutation-associated breast cancers (53) and may potentially be active in a subset of HR deficient tumors. Preclinical studies show DNA damage promotes neoantigen expression. Thus, it is postulated that PARP inhibitor induced tumor mutational burden could increase neoantigen expression thereby improving immune-tumor recognition. The combination of PARP inhibition and PD1/PD-L1 inhibitors is currently being evaluated. MEDIOLA (NCT02734004) is a multi-cohort phase 2 study which includes patients with germline BRCA mutated HER2-negative metastatic breast cancers treated with olaparib in combination with durvalumab. The Javelin Parp Medley phase 2 study (NCT03330405) evaluates avelumab plus talazoparib in a cohort of TNBC and hormone receptor positive breast cancer patients and atezolizumab is currently being evaluated in combination with veliparib in patients with TNBC in a randomized phase 2 study (NCT02849496).
Figure 1 highlights other notable targeted therapy partners including MEK inhibitors, HDAC inhibitors and CDK inhibitors which are being evaluated in early phase trials in combination with PD1/PD-L1 inhibitors.
Immunotherapy combinations
CTLA-4 and PD-1 immune checkpoint pathways regulate T cells at different stages of the immune response. CTLA-4 is confined to T cells and is inhibitory at the initial priming phase of naïve T cell activation whilst PD-1 is more broadly expressed on activated T cells, B cells or myeloid cells and inhibitory at a later effector phase. Dual targeting can augment anti-tumor activity of monotherapy checkpoint inhibitors. In advanced melanoma, the combination of nivolumab and ipilimumab resulted in longer PFS (11.5 vs. 2.9 months, HR 0.43; 95% CI: 0.35–0.52), higher ORR [58% vs. 19%, odds ratio (OR) 6.46; 95% CI: 4.45–9.35] and OS [not reached (NR) versus 19.9 months, HR 0.55; 95% CI: 0.45–0.69) as compared to ipilimumab monotherapy (54). The phase 1/2 study of this combination (NCT01928394) includes a TNBC cohort, the results of which have yet to be reported. The single arm study of durvalumab in combination with tremelimumab was presented at ASCO 2017. Using the Simon two-stage design, 18 patients with metastatic breast cancer (MBC) were enrolled in the first stage, seven TNBC and 11 ER-positive. The ORR was 17% not meeting the criteria to proceed to the second stage. Of note, five out of seven (71%) patients with TNBC had clinical benefit to the combination (Santa-Maria et al., ASCO 2017). Other notable partnering immune-modulating agents are listed in Figure 1 and include: inhibitors of other immune checkpoints such as Tim-3. Lag-3, costimulatory molecules such as 4-1BB, glucocorticoid-induced TNFR (GITR), OX40, IDO inhibitor and cytokine targeting antibodies.
Radiation therapy in combination with immunotherapy
Lastly, local therapy with radiation can synergize with immunotherapy and augment local as well as distant (abscopal) antitumor effects through increasing the release of TAA, increase APC, improve DC function as well as enhance T cell priming (55). Ongoing phase 2 studies evaluate the safety and efficacy of concurrent pembrolizumab with palliative radiotherapy (RT) in patients with metastatic TNBC (NCT02730130) and ER-positive HER2-negative breast cancers (NCT03051672). The combination of radiotherapy and pembrolizumab was reported by Dr. Heather McArthur et al. at the European Society of Medical Oncology (ESMO) annual meeting 2017. Seventeen heavily pre-treated women with mTNBC requiring RT to 1 measurable site of metastatic disease were enrolled however a significant proportion of women were not evaluable at 13 weeks illustrating the poor prognosis of this population of patients. The combination was well tolerated. Out of the 6 evaluable patients, 2 (33%) showed responses outside of the RT field (McArthur et al. ESMO 2017).
Adoptive T cell therapy
Chimeric antigen receptor T (CAR-T) cell therapy is an exciting and developing field in immunotherapy for solid organ malignancies. CARs are synthetic receptors derived against predetermined TAAs and transfected into autologous T cells which then recognize TAAs independent of MHC (56,57). The second and third generations of CARs have an additional costimulatory domain providing the abovementioned second signal which contributes to expansion, antitumor activity and cytokine secretion (56,57). CAR T-cell therapy targeting the CD19 antigen is furthest along in development and has been approved by the FDA for B-cell acute lymphoblastic leukaemia following remarkable responses seen in the phase 2 trial in pediatric and young adult patients (58). In breast cancer, CAR-T cell therapy is still early in development. Target antigens being studied in breast cancer include HER2 (NCT02547961, NCT02713984), CEA (NCT02349724) and mesothelin (NCT02792114) among others.
The future
Although an increasing number of trials of immunotherapy in breast cancer are being registered, there is at present no FDA approval for the use of immunotherapy in breast cancer as there are in other malignancies. With reports of sustained and durable responses achieved in the minority of breast cancer patients treated with immune therapy, the development of this treatment strategy should remain one of high priority.
Acknowledgements
None.
Footnote
Conflicts of Interest: The authors have no conflicts of interest to declare.
References
- Schreiber RD, Old LJ, Smyth MJ. Cancer Immunoediting: Integrating Immunity’s Roles in Cancer Suppression and Promotion. Science 2011;331:1565-70. [Crossref] [PubMed]
- Hanahan D, Weinberg RA. Hallmarks of Cancer: The Next Generation. Cell 2011;144:646-74. [Crossref] [PubMed]
- Klapper JA, Downey SG, Smith FO, et al. High-dose interleukin-2 for the treatment of metastatic renal cell carcinoma: A retrospective analysis of response and survival in patients treated in the Surgery Branch at the National Cancer Institute between 1986 and 2006. Cancer 2008;113:293-301. [Crossref] [PubMed]
- Atkins MB, Lotze MT, Dutcher JP, et al. High-Dose Recombinant Interleukin 2 Therapy for Patients With Metastatic Melanoma : Analysis of 270 Patients Treated Between 1985 and 1993. J Clin Oncol 1999;17:2105-16. [Crossref] [PubMed]
- Tivol EA, Borriello F, Schweitzer AN, et al. Loss of CTLA-4 leads to massive lymphoproliferation and fatal multiorgan tissue destruction, revealing a critical negative regulatory role of CTLA-4. Immunity 1995;3:541-7. [Crossref] [PubMed]
- Keir ME, Francisco LM, Sharpe AH. PD-1 and its ligands in T-cell immunity. Curr Opin Immunol 2007;19:309-14. [Crossref] [PubMed]
- Coulie PG, Van Den Eynde BJ, Van Der Bruggen P, et al. Tumour antigens recognized by T lymphocytes: At the core of cancer immunotherapy. Nat Rev Cancer 2014;14:135-46. [Crossref] [PubMed]
- Chen DS, Mellman I. Elements of cancer immunity and the cancer-immune set point. Nature 2017;541:321-30. [Crossref] [PubMed]
- Raval RR, Sharabi AB, Walker AJ, et al. Tumor immunology and cancer immunotherapy: Summary of the 2014 SITC primer. J Immunother Cancer 2014;2:14. [Crossref] [PubMed]
- Wolchok JD, Saenger Y. The Mechanism of Anti-CTLA-4 Activity and the Negative Regulation of T-Cell Activation. Oncologist 2008;13:2-9. [Crossref] [PubMed]
- Wang M, Zhang C, Song Y, et al. Mechanism of immune evasion in breast cancer. Onco Targets Ther 2017;10:1561-73. [Crossref] [PubMed]
- Syn NL, Teng MWL, Mok TSK, et al. De-novo and acquired resistance to immune checkpoint targeting. Lancet Oncol 2017;18:e731-41. [Crossref] [PubMed]
- Chin Y, Janseens J, Vandepitte J, et al. Phenotypic analysis of tumor-infiltrating lymphocytes from human breast cancer. Anticancer Res 1992;12:1463-6. [PubMed]
- Underwood JC. Lymphoreticular infiltration in human tumours: prognostic and biological implications: a review. Br J Cancer 1974;30:538-48. [Crossref] [PubMed]
- Aaltomaa S, Lipponen P, Eskelinen M, et al. Lymphocyte infiltrates as a prognostic variable in female breast cancer. Eur J Cancer 1992;28A:859-64. [Crossref] [PubMed]
- Loi S, Sirtaine N, Piette F, et al. Prognostic and predictive value of tumor-infiltrating lymphocytes in a phase III randomized adjuvant breast cancer trial in node-positive breast cancer comparing the addition of docetaxel to doxorubicin with doxorubicin-based chemotherapy: BIG 02-98. J Clin Oncol 2013;31:860-7. [Crossref] [PubMed]
- Loi S, Michiels S, Salgado R, et al. Tumor infiltrating lymphocytes are prognostic in triple negative breast cancer and predictive for trastuzumab benefit in early breast cancer: results from the FinHER trial. Ann Oncol 2014;25:1544-50. [Crossref] [PubMed]
- Adams S, Gray RJ, Demaria S, et al. Prognostic Value of Tumor-Infiltrating Lymphocytes in Triple-Negative Breast Cancers From Two Phase III Randomized Adjuvant Breast Cancer Trials: ECOG 2197 and ECOG 1199. J Clin Oncol 2014;32:2959-66. [Crossref] [PubMed]
- Luen SJ, Salgado R, Fox S, et al. Tumour-infiltrating lymphocytes in advanced HER2-positive breast cancer treated with pertuzumab or placebo in addition to trastuzumab and docetaxel: a retrospective analysis of the CLEOPATRA study. Lancet Oncol 2017;18:52-62. [Crossref] [PubMed]
- Shurin MR. Dual role of immunomodulation by anticancer chemotherapy. Nat Med 2013;19:20-2. [Crossref] [PubMed]
- Perou CM, Sørlie T, Eisen MB, et al. Molecular portraits of human breast tumours. Nature 2000;406:747-52. [Crossref] [PubMed]
- van de Vijver MJ, He YD, et al. A gene-expression signature as a predictor of survival in breast cancer. N Engl J Med 2002;347:1999-2009. [Crossref] [PubMed]
- Burstein MD, Tsimelzon A, Poage GM, et al. Comprehensive Genomic Analysis Identifies Novel Subtypes and Targets of Triple-negative Breast Cancer. Clin Cancer Res 2015;21:1688-98. [Crossref] [PubMed]
- Desmedt C, Haibe-Kains B, Wirapati P, et al. Biological processes associated with breast cancer clinical outcome depend on the molecular subtypes. Clin Cancer Res 2008;14:5158-65. [Crossref] [PubMed]
- Lehmann BD, Bauer JA, Chen X, et al. Identification of human triple-negative breast cancer subtypes and preclinical models for selection of targeted therapies. J Clin Invest 2011;121:2750-67. [Crossref] [PubMed]
- Lehmann BD, Jovanović B, Chen X, et al. Refinement of Triple-Negative Breast Cancer Molecular Subtypes: Implications for Neoadjuvant Chemotherapy Selection. PLoS One 2016;11:e0157368. [Crossref] [PubMed]
- Doherty MR, Cheon H, Junk DJ, et al. Interferon-beta represses cancer stem cell properties in triple-negative breast cancer. Proc Natl Acad Sci 2017;114:13792-7. [Crossref] [PubMed]
- Mittendorf E A, Philips A V, Meric-Bernstam F, et al. PD-L1 expression in triple-negative breast cancer. Cancer Immunol Res 2014;2:361-70. [Crossref] [PubMed]
- Ghebeh H, Mohammed S, Al-Omair A, et al. The B7-H1 (PD-L1) T Lymphocyte-Inhibitory Molecule Is Expressed in Breast Cancer Patients with Infiltrating Ductal Carcinoma: Correlation with Important High-Risk Prognostic Factors. Neoplasia 2006;8:190-8. [Crossref] [PubMed]
- Castle JC, Kreiter S, Diekmann J, et al. Exploiting the mutanome for tumor vaccination. Cancer Res 2012;72:1081-91. [Crossref] [PubMed]
- Schumacher TN, Schreiber RD. Neoantigens in cancer immunotherapy. Science 2015;348:69-74. [Crossref] [PubMed]
- Snyder A, Makarov V, Merghoub T, et al. Genetic Basis for Clinical Response to CTLA-4 Blockade in Melanoma. N Engl J Med 2014;371:2189-99. [Crossref] [PubMed]
- Rizvi NA, Hellmann MD, Snyder A, et al. Mutational landscape determines sensitivity to PD-1 blockade in non-small cell lung cancer. Science 2015;348:124-8. [Crossref] [PubMed]
- Cancer Genome Atlas Network. Comprehensive molecular portraits of human breast tumours. Nature 2012;490:61-70. [Crossref] [PubMed]
- Nik-Zainal S, Davies H, Staaf J, et al. Landscape of somatic mutations in 560 breast cancer whole-genome sequences. Nature 2016;534:47-54. [Crossref] [PubMed]
- Haricharan S, Bainbridge MN, Scheet P, et al. Somatic mutation load of estrogen receptor-positive breast tumors predicts overall survival: An analysis of genome sequence data. Breast Cancer Res Treat 2014;146:211-20. [Crossref] [PubMed]
- Campeau PM, Foulkes WD, Tischkowitz MD. Hereditary breast cancer: New genetic developments, new therapeutic avenues. Hum Genet 2008;124:31-42. [Crossref] [PubMed]
- Kraya AA, Maxwell KN, Wenz BM, et al. Abstract 4511: Characterization of tumor neoantigen repertoire in patients with germline BRCA1/2 mutations. Cancer Res 2016;76:abstr 4511.
- Davies H, Glodzik D, Morganella S, et al. HRDetect is a predictor of BRCA1 and BRCA2 deficiency based on mutational signatures. Nat Med 2017;23:517-25. [Crossref] [PubMed]
- Zhao EY, Shen Y, Pleasance E, et al. Homologous Recombination Deficiency and Platinum-Based Therapy Outcomes in Advanced Breast Cancer. Clin Cancer Res 2017;23:7521-30. [Crossref] [PubMed]
- Peoples GE, Holmes JP, Hueman MT, et al. Combined clinical trial results of a HER2/neu (E75) vaccine for the prevention of recurrence in high-risk breast cancer patients: U.S. Military Cancer Institute Clinical Trials Group study I-01 and I-02. Clin Cancer Res 2008;14:797-803. [Crossref] [PubMed]
- Mittendorf EA, Clifton GT, Holmes JP, et al. Clinical trial results of the HER-2/neu (E75) vaccine to prevent breast cancer recurrence in high-risk patients: from US Military Cancer Institute Clinical Trials Group Study I-01 and I-02. Cancer 2012;118:2594-602. [Crossref] [PubMed]
- Le DT, Pardoll DM, Jaffee EM. Cellular vaccine approaches. Cancer J 2010;16:304-10. [Crossref] [PubMed]
- Czerniecki BJ, Roses RE, Koski GK. Development of vaccines for high-risk ductal carcinoma in situ of the breast. Cancer Res 2007;67:6531-4. [Crossref] [PubMed]
- Lowenfeld L, Mick R, Datta J, et al. Dendritic cell vaccination enhances immune responses and induces regression of HER2pos DCIS independent of route: Results of randomized selection design trial. Clin Cancer Res 2017;23:2961-71. [Crossref] [PubMed]
- Hodi FS, O’Day SJ, McDermott DF, et al. Improved survival with ipilimumab in patients with metastatic melanoma. N Engl J Med 2010;363:711-23. [Crossref] [PubMed]
- Vonderheide RH, Lorusso PM, Khalil M, et al. Tremelimumab in combination with exemestane in patients with advanced breast cancer and treatment-associated modulation of inducible costimulator expression on patient T cells. Clin Cancer Res 2010;16:3485-94. [Crossref] [PubMed]
- Nanda R, Chow LQ, Dees EC, et al. Pembrolizumab in patients with advanced triple-negative breast cancer: Phase Ib keynote-012 study. J Clin Oncol 2016;34:2460-7. [Crossref] [PubMed]
- O’Shaughnessy J, Schwartzberg L, Danso MA, et al. Phase III Study of Iniparib Plus Gemcitabine and Carboplatin Versus Gemcitabine and Carboplatin in Patients With Metastatic Triple-Negative Breast Cancer. J Clin Oncol 2014;32:3840-7. [Crossref] [PubMed]
- Dirix LY, Takacs I, Jerusalem G, et al. Avelumab, an anti-PD-L1 antibody, in patients with locally advanced or metastatic breast cancer: a phase 1b JAVELIN Solid Tumor study. Breast Cancer Res Treat 2018;167:671-86. [Crossref] [PubMed]
- Zitvogel L, Kepp O, Kroemer G. Immune parameters affecting the efficacy of chemotherapeutic regimens. Nat Rev Clin Oncol 2011;8:151-60. [Crossref] [PubMed]
- Park JW, Liu MC, Yee D, et al. Adaptive Randomization of Neratinib in Early Breast Cancer. N Engl J Med 2016;375:11-22. [Crossref] [PubMed]
- Robson M, Im SA, Senkus E, et al. Olaparib for Metastatic Breast Cancer in Patients with a Germline BRCA Mutation. N Engl J Med 2017;377:523-33. [Crossref] [PubMed]
- Wolchok JD, Chiarion-Sileni V, Gonzalez R, et al. Overall Survival with Combined Nivolumab and Ipilimumab in Advanced Melanoma. N Engl J Med 2017;377:1345-56. [Crossref] [PubMed]
- Weichselbaum RR, Liang H, Deng L, et al. Radiotherapy and immunotherapy: a beneficial liaison? Nat Rev Clin Oncol 2017;14:365-79. [Crossref] [PubMed]
- Yeku O, Li X, Brentjens RJ. Adoptive T-Cell Therapy for Solid Tumors. Am Soc Clin Oncol Educ Book 2017;37:193-204. [Crossref] [PubMed]
- Yu S, Li A, Liu Q, et al. Chimeric antigen receptor T cells: a novel therapy for solid tumors. J Hematol Oncol 2017;10:78. [Crossref] [PubMed]
- Maude SL, Pulsipher MA, Boyer MW, et al. Efficacy and Safety of CTL019 in the First US Phase II Multicenter Trial in Pediatric Relapsed/Refractory Acute Lymphoblastic Leukemia: Results of an Interim Analysis. Blood 2016;128:2801.