Postoperative stereotactic body radiotherapy for spinal metastases
Introduction
Stereotactic body radiotherapy (SBRT) is an emerging treatment for spinal metastases. It has been defined as “The precise delivery of highly conformal and image-guided hypofractionated external beam radiotherapy, delivered in a single or few fraction(s), to an extracranial body target with doses at least biologically equivalent to a radical course when given over a protracted conventionally (1.8–3.0 Gy/fraction) fractionated schedule” (1). Fundamentally, SBRT aims to deliver with extreme precision and accuracy an ablative dose of radiation to the diseased vertebral segment while maintaining a safe dose tolerance to the surrounding critical structures (i.e., spinal cord, esophagus). The published clinical reports have established its feasibility, safety and efficacy in achieving local control, however, the data consist of retrospective single institution series, multi-institutional pooled data and a few prospective studies. Currently, two international ongoing phase II/III trials [NCT00922974 and NCT02512965 (2)] for intact spinal metastases are comparing spine SBRT to conventional radiation therapy (CRT) in patients with no prior surgery and radiation exposure. These trials are intended to prove whether spine SBRT yields superior pain control with respect to complete pain response rates, and imaging-based local control as compared to CRT. Currently, there are no prospective randomized trials evaluating SBRT as a salvage therapy for CRT failures or its role in the post-operative patient with spine metastases.
The focus of this review is on SBRT as an alternative to CRT for patients with spinal metastases that have undergone surgery. Those early adopters of post-operative SBRT argue that if we put a patient through a major operation we should deliver an effective adjuvant therapy intended to maximize local control. The intent of the overall procedure is to decompress the critical neural structures, stabilize and control the spinal metastases that have demonstrated biologic aggressiveness such that an operation was warranted. Post-op SBRT is not without technical challenges and this is likely why it is still at the emerging stage of global adoption. The challenges include imaging distortions secondary to the surgical hardware, concerns on the impact of the surgical hardware on the dosimetry and if post-op SBRT would cause wound dehiscence or hardware failure. Although the literature is limited, much has been learned and, in this review, we provide a summary of the clinical and technical aspects of postoperative SBRT for spinal metastases to guide safe practice.
Surgical management
The surgical intent for spinal metastases traditionally involves thecal sac decompression, stabilization with instrumentation or cement packing and tumor debulking. Indications for surgery include symptomatic pathological compression fracture, mechanical instability which can be assessed using Spinal Instability Neoplastic Score (SINS) (3) and symptomatic single level malignant epidural spinal cord compression (MESCC). In order to describe the extent of epidural disease, with respect to its anatomic relation to the spinal cord on MRI and any clinical neurological motor deficits, the Bilsky grading system (4) and the American Spinal Injury Association (ASIA) classification system (5) are common assessment tools, respectively. The SINS, Bilsky and ASIA classifications are summarized in Tables 1, 2, and 3, respectively.
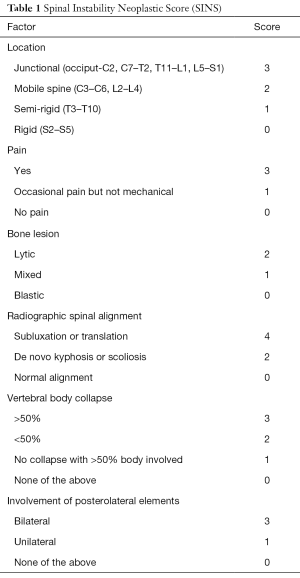
Full table
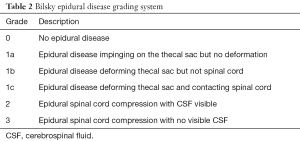
Full table
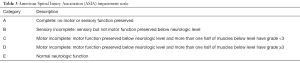
Full table
The role of surgery followed by palliative CRT for symptomatic single level MESCC is supported by level I evidence (6). Surgery was shown to improve ambulatory status, pain control and overall survival compared to palliative CRT alone (30 Gy in 10 fractions) in the Patchell Phase III randomized controlled trial. It is important to note that patients in this study were highly selected and, more commonly in practice, the population in question are patients who present with multi-level MESCC, where surgery is considered to be too invasive, and palliative CRT has been the default treatment of choice. Recently, surgical innovations have been developed to reduce the morbidity of traditional open invasive surgery. These include percutaneous instrumentation, cement augmentation procedures and minimally invasive decompression. The aim with these techniques is to minimize the wound created; for example, the tubular retraction system that allows for epidural disease resection is only 2 cm and it has been shown by Massicotte et al. that the median time to SBRT was 6.5 days (7). This represents a major advance as compared to the traditional 3 to 4 weeks for wound healing with open invasive instrumentation procedures. MR-guided spinal laser interstitial thermal therapy is an innovative minimally invasive technology being applied to the management of epidural disease. The aim is thermal ablation of epidural disease followed by SBRT as described by Tatsui et al. (8).
With respect to stabilization, newer percutaneous techniques have been developed. For example, use of cement augmentation procedures have been shown to be beneficial in reducing pain, opioid use and disability scores in patients with pathologic vertebral compression fracture (VCF) in a randomized trial (9) and, for more complex cases, percutaneous instrumentation has been shown to be a convenient way to stabilize with hardware without the morbidity of open invasive surgical instrumentation. There is always a role for open invasive procedures but care must be taken in cancer patients to consider less invasive surgery given that life expectancy is often limited and interruptions should be minimized in the overall cancer care.
With respect to epidural decompression, controversy exists on the role of surgical debulking of low grade epidural disease. Certainly, as shown by Patchell et al. (6), when high grade epidural disease causes symptomatic MESCC then surgery is warranted and beneficial. However, for the asymptomatic high-grade epidural disease patient, or even low grade epidural disease in selected patients, resection may have a role as it has been shown to improve SBRT outcomes. Al-Omair et al. reported that the downgrading of epidural disease has a therapeutic benefit with respect to local control following SBRT (10). The local control rates at one year for patients with pre-operative high-grade epidural disease (Bilsky 2 or 3) that was downgraded to 0 or 1 was 95%, as compared to 50% if no downgrading occurred after surgery (P=0.0009) (10). Fundamentally, it has consistently been shown that failure post-SBRT is most common within the epidural space. This pattern of failure may be explained by the inherent underdosing required to respect the adjacent spinal cord tolerance, biologic aggressiveness of the disease, or geographic miss associated with conformality of the dose distribution. Ultimately, the management of epidural disease with minimally invasive techniques is increasingly becoming an area of research and innovation.
Conventional postoperative external beam radiotherapy
Following surgical treatment, postoperative CRT is most commonly employed delivering what is considered a palliative dose of 20 Gy in 5 fractions or 30 Gy in 10 fractions (6,11-14). The interpretation of CRT outcomes in the literature is challenging for many reasons including the heterogeneity of the reported endpoints. Although many series report pain and neurologic function, these endpoints may not be specific surrogates for local control, as postoperative pain is not uncommonly encountered in these patients. Ambulation is another reported endpoint, including in the Patchell phase III randomized trial (6). Again, this endpoint is rather complex and does not necessarily reflect disease control. Furthermore, it is subjective in nature in that there is no standardized outcome assessment tool for ambulation. In our opinion, imaging-based local control is the endpoint of interest and more specifically with respect to justifying a transition to SBRT. A recent review of the literature identified only 3 small retrospective series that included few patients followed with various imaging modalities (11,13,14). Overall, the crude rate of local control using postoperative CRT ranged from 4% to 79% (15).
Radiation fields of CRT typically encompass the preoperatively involved vertebrae with one vertebral body above and below. Hardware at the level of preoperative disease in usually included in the standard parallel opposed anteroposterior fields. A single posterior field can also be used if the patient anatomy allows for acceptable coverage but is discouraged due to excessive skin dose which is essentially the surgical wound, and the underdosing of the anterior vertebral body. The main advantages of CRT lie in that patient set-up is quick and less labor intensive with respect to planning and delivery when compared to SBRT.
Postoperative SBRT
Reported clinical series of postoperative SBRT indicate at least comparable if not better local control as compared to CRT series (15), with a 1-year local control rate of approximately 80–90% (16). Unlike CRT series, SBRT studies report MRI-based local control as the primary endpoint and tend to include more radio-resistant tumors and/or patients with a history of prior CRT. Therefore, the high rates of local control are likely reflecting superior outcomes as compared to CRT, but no randomized trials have been conducted. Table 4 summarizes those selected SBRT studies specific to postoperative spinal metastases with at least 20 patients in the series and procedures beyond simple cement augmentation as the primary surgical technique.
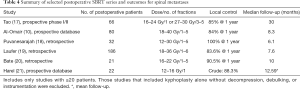
Full table
Risk factors, patterns and assessment of disease local recurrence
A higher grade of postoperative epidural disease (Bilsky 2 or 3 vs. 0 or 1) and lower prescribed dose per fraction (18–40 Gy in 3–5 fractions vs. 18–26 Gy in 1 or 2) have been shown to be predictive for local recurrence following postoperative SBRT in the series from Al-Omair et al. (10). Similarly, Laufer et al. reported higher rates of local control when treating with high dose single fraction and hypofractionated SBRT as compared to lower dose hypofractionated SBRT (19). It may be that the hypoxic environment of the post-operative tissue bed requires greater SBRT biologically effective doses, but as yet there is no high level evidence to support conclusively one SBRT fractionation scheme over another. In addition, there have been no robust dosimetric parameters found to predict local control as a means to guide treatment planning. The overall intent is to respect the tolerance to the organs-at-risk while maximizing coverage of the planning target volume.
With respect to patterns of failure, it has been observed that the epidural space is the most common anatomic location of tumor recurrence, and this is similar to that observed for intact metastases treated with SBRT. Importantly, marginal and adjacent level failures have not been commonly observed, and this is critical as the clinical target volume (CTV) in the post-operative patient is significantly tailored as compared to what we traditionally treated with CRT. This observation also likely reflects the tendency to be generous with the CTV and encompassing the surgical bed as opposed to only where the tumor was thought to be present pre-operatively.
Chan et al. reported detailed patterns of failure analysis specific to patients treated with post-operative SBRT (22). They divided patients into those with pre- and post-operative anterior-only epidural disease and those with anterior and posterior epidural disease, and then subdivided the spinal canal into 6 sectors based on the International Spine Radiosurgery Consortium (ISRC) anatomic classification system (22). The first major observation from that study was to respect the pre-operative location of epidural tumor in the post-operative CTV. This recommendation was based on the pre-operative location of epidural disease predicting failure as opposed to the post-operation location of the epidural disease. Second, for those patients with pre-operative anterior confined epidural disease, subsequent failure could occur within the anteriorly epidural sector but also in the anterio-lateral sectors. In those patients with pre-operative circumferential disease, failure could occur in any of the anterior or posterior sectors. This led to recommendations of CTV delineation specific to the post-operative SBRT patient which have been further refined based on a group of experts by Redmond et al. to guide safe target volume delineation (23).
The interpretation of post-SBRT imaging-based response is also a major challenge. For example, osseous pseudo-progression on early post-SBRT MRI T1 and T2 sequences has been recently described and observed to occur in ~30% of the patients between 3–6 months post-SBRT, and this is not observed with CRT (24). This reflects the lack of understanding of signal changes in the bone associated with high dose radiation. For example, within the bone of the treated spinal segment a darkening in the T1 signal is not uncommonly observed, and this can be reported as progression if the radiologist is unaware of the treatment. Cruz et al. reported such a case where pathologic confirmation of radiation fibrosis and necrosis was observed as opposed to tumor progression, which represented one of the first reports to highlight the issue (25). The SPine response assessment in Neuro-Oncology (SPINO) group reported early recommendations regarding post-SBRT imaging interpretation which consist of using MRI for tumor response assessment, interpretation by a neuro-radiologist and radiation oncologist in equivocal cases, defining local control as the absence of progression in the treated area based on serial imaging, and defining local progression as unequivocal disease progression on serial imaging or new/progressing epidural disease (26). The same group recommended against applying the commonly used RECIST criteria in assessing response post-SBRT as RECIST was not developed for bone metastases and was based on linear dimensions (26).
Postoperative SBRT volume definitions, dose constraints and technique
Consensus contouring guidelines for postoperative SBRT were recently reported to standardize volume delineation to guide safe practice (23). Briefly, gross tumor volume (GTV) was defined as residual disease visible on MRI and CT, and CTV the margin applied to encompass the surgical bed with care to encompass the preoperative extent of the disease in bone, epidural and paraspinal tissue regardless of the extent of resection. Adjacent anatomical compartments (ISRC sectors) (22) at risk of microscopic disease are also part of the CTV. At the Sunnybrook Odette Cancer Centre of the University of Toronto, Toronto, Ontario, Canada, we also add a 5-mm craniocaudal expansion on epidural disease in order to ensure that the potential for geographic miss is minimized in this axis. We then subtract the CTV from the spinal cord and/or thecal sac to create a final CTV, and thereby maximizing the dose to the epidural space in all cases. An expansion of 2 mm on the final CTV is used to create a PTV as shown in the example case (Figure 1). We use the same PTV margin for treating longer target volumes (i.e., three vertebral levels or more) as our published data indicated that 2 mm is an adequate margin for set-up uncertainty in these patients (27).
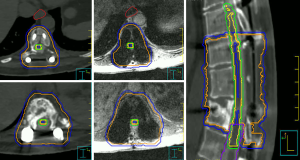
For treatment planning, volumetric thin slice T1 and T2 weighted non-contrast axial MRIs are acquired at least one vertebral body above and below the target spinal segment and fused to the thin slice CT simulation scan. Gadolinium may be useful for paraspinal disease characterization and CT myelogram may be required if the metallic artifact is severe such that the MRI is distorted or blacked out. The pre-operative MRI can also be fused if feasible but must be examined to ensure that all areas of pre-operative disease are encompassed in the CTV. The spinal cord/thecal sac is usually contoured on T1 weighted axial MRI and the axial T2 can help in particular in regions with metallic artifact, and/or based on the CT myelogram. We apply a 1.5 mm expansion on the spinal cord as a planning organ at risk volume (PRV), but for the thecal sac no PRV is applied. We also use the same PRV margin for longer target volumes based on our published data (27). Overlapping cord and thecal sac contours at T12 to L1 is considered a safe practice. Nerve roots can also be contoured especially in the sacral area with a restriction of no hot spots.
In addition to metal artifact, titanium hardware has an impact on dose distribution including underestimation of the dose in front of the hardware by 6% and underdosing of areas beyond the hardware by 7% (15). In order to minimize these effects, one should keep the weight of the beams passing through the hardware as low as possible. Furthermore, we contour the metal hardware and assign a density to account for the difference. In many cases, we are not provided with the information regarding the composition of the hardware. We contour the hardware using different views of CT (all three views as well as the DRRs) and assign it a density of titanium and also contour the image artifacts and assign it a density of water. Care is taken to contour a minimum amount of artifacts and not assign regions of bone with water.
Generally, doses of postoperative SBRT are similar to SBRT for intact spinal metastases, and there is no consensus on dose and fractionation (16). Our standard dose is 24 Gy in two fractions with a 17 Gy limit to the spinal cord PRV/thecal sac. In patients with prior CRT, we treat with 24 Gy in 2 fractions with a 12.2 Gy limit to the spinal cord PRV/thecal sac. For patients with 2 courses of prior CRT, prior SBRT or spinal segments involving 3 or more levels, we tend to treat with 30 Gy in 4 fractions and apply spinal cord constraints based on published guidelines by Sahgal et al. (28-31).
With respect to treatment, image guidance is critical and mandatory with cone-beam CT and/or stereoscopic imaging. We recommend a translational and rotational tolerance of 1mm and 1 degree, respectively, and 6-degree freedom positional correction at the level of the treatment couch or linac itself (i.e., Cyberknife) (32).
Toxicities following postoperative SBRT
Based on level III evidence, SBRT is considered a safe treatment when performed properly. Radiation myelopathy, plexopathy, and esophageal toxicities are rare (33). As the technique and dose tolerance to critical organs-at-risk have been largely established for safe practice, vertebral compression fracture is the adverse event of concern, and observed typically in 10–15% of patients. Risk factors include SBRT with ≥20 Gy/fraction, presence of a baseline VCF, spinal misalignment and lytic tumor (33-37). In some patients, the incidence can approach even 40% in particular when treating with 24 Gy in 1 fraction. In high risk patients, there is a role for prophylactic (pre-SBRT or immediately post-SBRT) stabilization with interventions such as percutaneous vertebroplasty (cement augmentation), percutaneous instrumentation or open surgical stabilization. Multidisciplinary discussion is essential to optimize treatment outcome in high risk patients.
Given that most postoperative patients have hardware stabilization in place prior to SBRT treatment, the issue of VCF may not be as clinically important as in the case of intact metastases treated with SBRT. In a cohort of 80 patients who were treated with postoperative SBRT, Al-Omair et al. reported 9 cases of VCF (5 new and 4 progression) (10). In the same cohort, only one had hardware failure post SBRT and no myelopathy or neuropathy observed. It is important to note that although fracture occurs, most settle with only 20-30% being salvaged with typically a cement augmentation procedure (38). The impact of SBRT on instrumentation failure was addressed by Harel et al. (39). In that study, a lower rate of instrumentation failure was observed with SBRT compared to CRT (0% vs. 43%). In addition, authors found a higher rate of fusion with SBRT (50% vs. 17%). The same group assessed the effect of SBRT on wound healing and found no significant wound healing complications at a mean follow-up of 12.5 months following postoperative SBRT (21).
Example case
A 58-year-old patient with metastatic breast cancer treated with 24 Gy in a single fraction to T12. Four years later, the patient developed a local recurrence at T12 and was re-treated with a second course of SBRT using 30 Gy in 4 fractions. She then presented after two years with progressive back pain and was found to have disease recurrence at T10, T11 and T12 with Bilsky 1C epidural extension (Figure 2). The patient underwent T10-T11 epidural tumour decompression, T9–T12 laminectomy, and T8-L1 instrumented fusion. Postoperatively, she received a 3rd course of SBRT to T10–T12. The prescribed dose was 30 Gy in 5 fractions which is our preferred third time re-treatment dose with SBRT. This patient remained locally controlled until her death 6 months later. Figure 3 shows how metal artifacts can make cord visualization very difficult and mimic residual disease on MRI, making the CT myelogram invaluable for accurate cord delineation in this example. Figures 1,4-6 show target contours, spinal cord and thecal sac contours, the treatment plan, and dose volume histogram, respectively, for this challenging case.
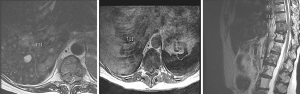
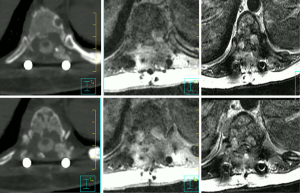
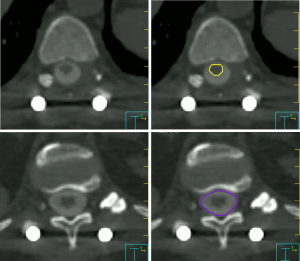
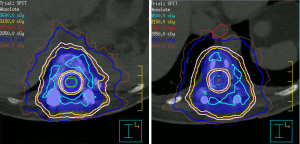
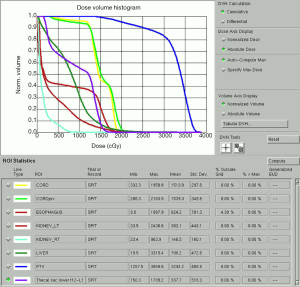
Conclusions
Postoperative SBRT for spinal metastases is increasing in clinical practice as an adjuvant treatment that is both safe and efficacious. Contouring guidelines represent a significant advance in standardizing the delineation of these complex targets. It is recognized that clinical data is limited to predominantly retrospective analyses of prospective databases and therefore, randomized trials are needed to confirm superiority over conventional external beam palliative radiotherapy, in addition to SBRT dose finding studies to determine the optimal postoperative SBRT regimen.
Acknowledgements
The authors acknowledge all the members of the CNS Multidisciplinary Oncology team at the Odette Cancer Centre/Sunnybrook Health Sciences Centre/University of Toronto for their support.
Footnote
Conflicts of Interest: Dr Arjun Sahgal has received honorarium and travel support for past educational seminars from Medtronic, Elekta AB, Accuray Inc., and Varian Medical Systems. He also holds research grants with Elekta AB. Dr Victor Yang owns stock and royalty interests in 7D Surgical. The other authors have no conflicts of interest to declare.
References
- Sahgal A, Roberge D, Schellenberg D, et al. The Canadian Association of Radiation Oncology scope of practice guidelines for lung, liver and spine stereotactic body radiotherapy. Clin Oncol (R Coll Radiol) 2012;24:629-39. [Crossref] [PubMed]
- Sahgal A, Myrehaug S, Dennis K, et al. A randomized phase II/III study comparing stereotactic body radiotherapy (SBRT) versus conventional palliative radiotherapy (CRT) for patients with spinal metastases (NCT02512965). J Clin Oncol 2017;35:abstr TPS10129.
- Fisher CG, DiPaola CP, Ryken TC, et al. A novel classification system for spinal instability in neoplastic disease: an evidence-based approach and expert consensus from the Spine Oncology Study Group. Spine (Phila Pa 1976) 2010;35:E1221-9. [Crossref] [PubMed]
- Bilsky MH, Laufer I, Fourney DR, et al. Reliability analysis of the epidural spinal cord compression scale. J Neurosurg Spine 2010;13:324-8. [Crossref] [PubMed]
- Kirshblum SC, Burns SP, Biering-Sorensen F, et al. International standards for neurological classification of spinal cord injury (revised 2011). J Spinal Cord Med 2011;34:535-46. [Crossref] [PubMed]
- Patchell RA, Tibbs PA, Regine WF, et al. Direct decompressive surgical resection in the treatment of spinal cord compression caused by metastatic cancer: a randomised trial. Lancet 2005;366:643-8. [Crossref] [PubMed]
- Massicotte E, Foote M, Reddy R, et al. Minimal access spine surgery (MASS) for decompression and stabilization performed as an out-patient procedure for metastatic spinal tumours followed by spine stereotactic body radiotherapy (SBRT): first report of technique and preliminary outcomes. Technol Cancer Res Treat 2012;11:15-25. [Crossref] [PubMed]
- Tatsui CE, Lee SH, Amini B, et al. Spinal Laser Interstitial Thermal Therapy: A Novel Alternative to Surgery for Metastatic Epidural Spinal Cord Compression. Neurosurgery 2016;79 Suppl 1:S73-S82. [Crossref] [PubMed]
- Berenson J, Pflugmacher R, Jarzem P, et al. Balloon kyphoplasty versus non-surgical fracture management for treatment of painful vertebral body compression fractures in patients with cancer: a multicentre, randomised controlled trial. Lancet Oncol 2011;12:225-35. [Crossref] [PubMed]
- Al-Omair A, Masucci L, Masson-Cote L, et al. Surgical resection of epidural disease improves local control following postoperative spine stereotactic body radiotherapy. Neuro Oncol 2013;15:1413-9. [Crossref] [PubMed]
- Epstein-Peterson ZD, Sullivan A, Krishnan M, et al. Postoperative radiation therapy for osseous metastasis: Outcomes and predictors of local failure. Pract Radiat Oncol 5:e531-6. [Crossref] [PubMed]
- Fehlings MG, Nater A, Tetreault L, et al. Survival and Clinical Outcomes in Surgically Treated Patients With Metastatic Epidural Spinal Cord Compression: Results of the Prospective Multicenter AOSpine Study. J Clin Oncol 2016;34:268-76. [Crossref] [PubMed]
- Sellin JN, Suki D, Harsh V, et al. Factors affecting survival in 43 consecutive patients after surgery for spinal metastases from thyroid carcinoma. J Neurosurg Spine 2015;23:419-28. [Crossref] [PubMed]
- Sellin JN, Gressot LV, Suki D, et al. Prognostic Factors Influencing the Outcome of 64 Consecutive Patients Undergoing Surgery for Metastatic Melanoma of the Spine. Neurosurgery 2015;77:386-93; discussion 393. [Crossref] [PubMed]
- Redmond KJ, Lo SS, Fisher C, et al. Postoperative Stereotactic Body Radiation Therapy (SBRT) for Spine Metastases: A Critical Review to Guide Practice. Int J Radiat Oncol Biol Phys 2016;95:1414-28. [Crossref] [PubMed]
- Huo M, Sahgal A, Pryor D, et al. Stereotactic spine radiosurgery: Review of safety and efficacy with respect to dose and fractionation. Surg Neurol Int 2017;8:30. [Crossref] [PubMed]
- Tao R, Bishop AJ, Brownlee Z, et al. Stereotactic Body Radiation Therapy for Spinal Metastases in the Postoperative Setting: A Secondary Analysis of Mature Phase 1-2 Trials. Int J Radiat Oncol Biol Phys 2016;95:1405-13. [Crossref] [PubMed]
- Puvanesarajah V, Lo SL, Aygun N, et al. Prognostic factors associated with pain palliation after spine stereotactic body radiation therapy. J Neurosurg Spine 2015.1-10. [PubMed]
- Laufer I, Iorgulescu JB, Chapman T, et al. Local disease control for spinal metastases following "separation surgery" and adjuvant hypofractionated or high-dose single-fraction stereotactic radiosurgery: outcome analysis in 186 patients. J Neurosurg Spine 2013;18:207-14. [Crossref] [PubMed]
- Bate BG, Khan NR, Kimball BY, et al. Stereotactic radiosurgery for spinal metastases with or without separation surgery. J Neurosurg Spine 2015;22:409-15. [Crossref] [PubMed]
- Harel R, Emch T, Chao S, et al. Quantitative Evaluation of Local Control and Wound Healing Following Surgery and Stereotactic Spine Radiosurgery for Spine Tumors. World Neurosurg 2016;87:48-54. [Crossref] [PubMed]
- Chan MW, Thibault I, Atenafu EG, et al. Patterns of epidural progression following postoperative spine stereotactic body radiotherapy: implications for clinical target volume delineation. J Neurosurg Spine 2016;24:652-9. [Crossref] [PubMed]
- Redmond KJ, Robertson S, Lo SS, et al. Consensus Contouring Guidelines for Postoperative Stereotactic Body Radiation Therapy for Metastatic Solid Tumor Malignancies to the Spine. Int J Radiat Oncol Biol Phys 2017;97:64-74. [Crossref] [PubMed]
- Amini B, Beaman CB, Madewell JE, et al. Osseous Pseudoprogression in Vertebral Bodies Treated with Stereotactic Radiosurgery: A Secondary Analysis of Prospective Phase I/II Clinical Trials. AJNR Am J Neuroradiol 2016;37:387-92. [Crossref] [PubMed]
- Cruz JP, Sahgal A, Whyne C, et al. Tumor extravasation following a cement augmentation procedure for vertebral compression fracture in metastatic spinal disease. J Neurosurg Spine 2014;21:372-7. [Crossref] [PubMed]
- Thibault I, Chang EL, Sheehan J, et al. Response assessment after stereotactic body radiotherapy for spinal metastasis: a report from the SPIne response assessment in Neuro-Oncology (SPINO) group. Lancet Oncol 2015;16:e595-603. [Crossref] [PubMed]
- Chang JH, Sangha A, Hyde D, et al. Positional Accuracy of Treating Multiple Versus Single Vertebral Metastases With Stereotactic Body Radiotherapy. Technol Cancer Res Treat 2017;16:231-7. [Crossref] [PubMed]
- Sahgal A, Weinberg V, Ma L, et al. Probabilities of radiation myelopathy specific to stereotactic body radiation therapy to guide safe practice. Int J Radiat Oncol Biol Phys 2013;85:341-7. [Crossref] [PubMed]
- Wong CS, Fehlings MG, Sahgal A. Pathobiology of radiation myelopathy and strategies to mitigate injury. Spinal Cord 2015;53:574-80. [Crossref] [PubMed]
- Sahgal A, Ma L, Gibbs I, et al. Spinal cord tolerance for stereotactic body radiotherapy. Int J Radiat Oncol Biol Phys 2010;77:548-53. [Crossref] [PubMed]
- Sahgal A, Ma L, Weinberg V, et al. Reirradiation human spinal cord tolerance for stereotactic body radiotherapy. Int J Radiat Oncol Biol Phys 2012;82:107-16. [Crossref] [PubMed]
- Hyde D, Lochray F, Korol R, et al. Spine stereotactic body radiotherapy utilizing cone-beam CT image-guidance with a robotic couch: intrafraction motion analysis accounting for all six degrees of freedom. Int J Radiat Oncol Biol Phys 2012;82:e555-62. [Crossref] [PubMed]
- Sahgal A, Whyne CM, Ma L, et al. Vertebral compression fracture after stereotactic body radiotherapy for spinal metastases. Lancet Oncol 2013;14:e310-20. [Crossref] [PubMed]
- Cunha MV, Al-Omair A, Atenafu EG, et al. Vertebral compression fracture (VCF) after spine stereotactic body radiation therapy (SBRT): analysis of predictive factors. Int J Radiat Oncol Biol Phys 2012;84:e343-9. [Crossref] [PubMed]
- Jawad MS, Fahim DK, Gerszten PC, et al. Vertebral compression fractures after stereotactic body radiation therapy: a large, multi-institutional, multinational evaluation. J Neurosurg Spine 2016;24:928-36. [Crossref] [PubMed]
- Boehling NS, Grosshans DR, Allen PK, et al. Vertebral compression fracture risk after stereotactic body radiotherapy for spinal metastases. J Neurosurg Spine 2012;16:379-86. [Crossref] [PubMed]
- Sahgal A, Atenafu EG, Chao S, et al. Vertebral compression fracture after spine stereotactic body radiotherapy: a multi-institutional analysis with a focus on radiation dose and the spinal instability neoplastic score. J Clin Oncol 2013;31:3426-31. [Crossref] [PubMed]
- Virk MS, Han JE, Reiner AS, et al. Frequency of symptomatic vertebral body compression fractures requiring intervention following single-fraction stereotactic radiosurgery for spinal metastases. Neurosurg Focus 2017;42:E8. [Crossref] [PubMed]
- Harel R, Chao S, Krishnaney A, et al. instrumentation failure after spine tumor resection and radiation: comparing conventional radiotherapy with stereotactic radiosurgery outcomes. World Neurosurg 2010;74:517-22. [Crossref] [PubMed]