Drug development in the era of precision medicine in oncology—Clues beyond randomization
The primary objective of any treatment in oncology is the improve patients’ overall survival (OS) and/or quality of life (QoL). Patients with solid tumors may often be cured thanks to local treatments including surgery and radiotherapy when then are free of distant metastases. In this setting, anticancer drugs may improve cure rates when combined to local treatments. In the recurrent and/or metastatic setting, drugs represent the main treatment option, while surgery and radiotherapy might still be used in a palliative intent in most cases. With the exception of germline tumors and lymphoma, drugs have a limited ability to cure patients in this setting, and patients most often need to receive sequential treatments for life.
In the ancestral paradigm of drug development in oncology, drugs used to be developed per cancer type following a well-established path that well suited chemotherapeutic agents for which antitumor activity largely depended on cancer types in preclinical models (Table 1). The first evaluation of new drugs in patients in phase I clinical trials was usually performed in patients who had exhausted standard of care, and not in healthy volunteers given the toxic nature of the drugs and their narrow therapeutic index. Dose escalation used to be open to patients with any type of cancer however, in order not to miss a serendipitous antitumor activity in unexpected cancer types. Phase I trials were not randomized, and enabled to establish the schedule and the recommended phase II dose that was the highest dose to be considered safe for further evaluation. Based on antitumor activity observed in preclinical models and during phase I trials, preliminary drug efficacy was then assessed in a specific cancer type and setting (usually in the recurrent and/or metastatic setting) in single-arm or randomized phase II clinical trials. Surrogate endpoints such as the objective response rate (ORR) or progression-free survival (PFS) were commonly used to have a rapid read-out of the efficacy. These surrogate endpoints were assessed by following over time the tumor burden, formerly the sum of the product of the two diameters of the target lesions (WHO criteria) (1), and more recently the sum of the largest diameter of up to five target lesions (RECIST) (2). Randomized phase III clinical trials were performed in a similar patient population in order to demonstrate an improvement in OS and/or QoL of the new treatment over the current standard of care. Randomization is the gold-standard approach for market access in order to avoid selection biases. Recently, seamless drug development led to increasingly replace phase II clinical trials with large expansion cohorts performed during phase I trials, in order to accelerate drug development (3). This paradigm based on the randomization for drug approval nicely fitted to drugs developed in specific but common cancer types, but is less suited to rare cancer types.

Full table
A better understanding of cancer biology led to the development of molecularly targeted agents (MTAs) that were specifically designed to modulate a molecular pathway in the tumor cells or their microenvironment, and immunotherapies that reactivate the immune system against cancer cells. Several MTAs, such as antiangiogenic agents or mammalian target of rapamycin (mTOR) inhibitors, were developed in specific cancer types without any molecular selection based on a biomarker, whereas the identification of molecular alterations in some cancer types formed the basis for a biomarker-driven drug development following a drug-diagnostic codevelopment (Table 1) (4). Most of MTAs developed with a companion diagnostic were initially tested in one single cancer type, such as crizotinib in anaplastic lymphoma kinase (ALK)-translocated non-small cell lung cancer (NSCLC) patients (5). The assumption that the predictive value of a molecular alteration in one cancer type would hold true in other cancer types led to the design of basket trials in which the selection was based on the occurrence of the molecular alteration in different cancer types (6-9). The example of vemurafenib in patients with BRAF V600E-mutated cancers however suggested that the predictive value of a molecular alteration might vary depending on the cancer type (6). Intratumor heterogeneity and coexisting molecular alterations might explain primary and secondary resistance to MTAs. Despites these caveats, MTAs have substantially improved the outcome of patients in several cancer types, although a minority of patients are eligible to these drugs (10).
Biomarker-driven drug development represents a challenge when the prevalence of the biomarker is low. In the pivotal phase III trial that compared crizotinib to chemotherapy in ALK-translocated NSCLC patients, 4,967 patients had to be screened in order to treat 347 patients (7%) (11). Umbrella trials were designed in specific cancer types in order to allow proposing different MTAs and/or immunotherapies depending on identified molecular alterations following a broad screening, with the advantage of being able to propose a treatment to all included patients (12). Overall, basket and umbrella trials are generally set up as simple parallel phase II clinical trials performed in histologically- and molecularly-defined subgroups of patients (13). They both accelerate drug development, although the ideal clinical trial would test simultaneously the efficacy of multiple drugs in multiple molecularly- and histologically-defined subgroups of patients. This trial however would unrealistically require tens of thousands of patients to evaluate the efficacy of each drug in each subgroup of patients with enough statistical power. Clinical trials that mix cancer types, molecular alterations and drugs have been conducted (14-19). These trials were actually not designed to assess the efficacy of any of the drugs tested in any subgroup of patients, but only to inform about the ability of the treatment algorithm used to allocate drugs to patients to improve their outcome (20). Treatment algorithms evaluated in these trials may be “marketed” in the same way than drugs, as did the company CureMatch® that was created following the results of the WINTHER trial (17,21).
The ancestral paradigm of drug development in oncology is further challenged by (I) the ever-expanding molecular segmentation of cancer with ever-smaller subgroups of patients who might benefit from specific MTAs or immunotherapies, and (II) the discovery of molecular alterations against which drugs may be effective across cancer types. A striking example is the NTRK gene fusions that are present in 0.3% of all cancer patients and efficiently targeted with NTRK inhibitors (22). While we strongly believe randomization should remain the gold-standard in large patient populations, novel ways of evaluating the efficacy of drugs are highly needed in small patient populations (Figure 1).
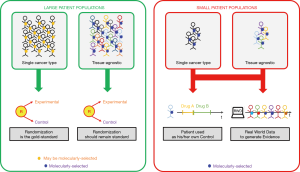
One approach is to use each patient as his/her own control by comparing the efficacy a drug to the efficacy of prior treatments received (14,16,17,23). The assumption made that tumor growth is linear over time is likely a fair approximation over a short period in the recurrent and/or metastatic setting, knowing that it takes decades for a cancer to become macroscopic. This approach avoids dealing with interpatient’ heterogeneity, especially for a tissue-agnostic drug development. The comparison of drugs’ efficacy in a same patient is however only valid if drug efficacy has been assessed using the same evaluation criteria (e.g., RECIST) and the same timing for tumor evaluations. The SHIVA02 clinical trial has been designed considering this by mandating to use the same tumor assessment method and timing during the two treatment periods (NCT03084757).
A second approach is the use of real-world data to generate evidence, by comparing the outcomes of treated and non-treated patient populations (24,25). Comparisons can be made using randomization and while adjusting on clinical and/or molecular parameters. Similar patient’s groups may also be selected using propensity score matching. This approach provides the additional advantage of representing a non-selected patient population as opposed to clinical trials. Major regulatory authorities, including the European Medicines Agency (EMA) and the United States Food and Drug Administration (FDA), have recognized the importance of real-world data as a source of complementary evidence for regulatory decisions (26,27). Many current retrospective real-world data efforts are however not of high enough quality to answer all outstanding questions. The key requirements indeed are to have a large, as heterogeneous as possible, patient population with curated and standardized clinical and molecular data. The quality of the data needs to be as high as the one collected in clinical trials, especially in terms of response to treatments received.
To conclude, there is an urgent need to develop new methods to foster drug development in the era of precision medicine, due the molecular segmentation of cancer and the tissue-agnostic predictive value of some molecular alterations. However, it is essential to keep in mind that local treatments, including surgery and radiotherapy, remain the main pillars of cancer therapy for which the ancestral paradigm per cancer type applies, and that randomization should still be considered the gold-standard approach to evaluate the efficacy of new treatments whenever feasible in large enough patient populations. For small patient populations, the use of each patient as his/her own control and real-world data represent appealing tools to generate robust evidence.
Acknowledgments
Funding: None.
Footnote
Provenance and Peer Review: This article was a standard submission to the journal. The article has undergone external peer review.
Conflicts of Interest: All authors have completed the ICMJE uniform disclosure form (available at http://dx.doi.org/10.21037/cco-21-29). CLT reports that he participated in advisory boards from BMS, MSD, Merck Serono, Astra Zeneca, Roche, Rakuten, Seattle Genetics, Roche, Nanobiotix. CLT serves as an unpaid editorial board member of Chinese Clinical Oncology from Aug 2019 to Jul 2021. The other authors have no conflicts of interest to declare.
Ethical Statement: The authors are accountable for all aspects of the work in ensuring that questions related to the accuracy or integrity of any part of the work are appropriately investigated and resolved.
Open Access Statement: This is an Open Access article distributed in accordance with the Creative Commons Attribution-NonCommercial-NoDerivs 4.0 International License (CC BY-NC-ND 4.0), which permits the non-commercial replication and distribution of the article with the strict proviso that no changes or edits are made and the original work is properly cited (including links to both the formal publication through the relevant DOI and the license). See: https://creativecommons.org/licenses/by-nc-nd/4.0/.
References
- Therasse P, Arbuck SG, Eisenhauer EA, et al. New guidelines to evaluate the response to treatment in solid tumors. European Organization for Research and Treatment of Cancer, National Cancer Institute of the United States, National Cancer Institute of Canada. J Natl Cancer Inst 2000;92:205-16. [Crossref] [PubMed]
- Eisenhauer EA, Therasse P, Bogaerts J, et al. New response evaluation criteria in solid tumours: revised RECIST guideline (version 1.1). Eur J Cancer 2009;45:228-47. [Crossref] [PubMed]
- Prowell TM, Theoret MR, Pazdur R. Seamless Oncology-Drug Development. N Engl J Med. 2016;374:2001-3. [Crossref] [PubMed]
- Jørgensen JT, Hersom M. Clinical and Regulatory Aspects of Companion Diagnostic Development in Oncology. Clin Pharmacol Ther 2018;103:999-1008. [Crossref] [PubMed]
- Kwak EL, Bang YJ, Camidge DR, et al. Anaplastic lymphoma kinase inhibition in non-small-cell lung cancer. N Engl J Med 2010;363:1693-1703. [Crossref] [PubMed]
- Hyman DM, Puzanov I, Subbiah V, et al. Vemurafenib in Multiple Nonmelanoma Cancers with BRAF V600 Mutations. N Engl J Med 2015;373:726-36. [Crossref] [PubMed]
- Drilon A, Laetsch TW, Kummar S, et al. Efficacy of Larotrectinib in TRK Fusion–Positive Cancers in Adults and Children. N Engl J Med 2018;378:731-9. [Crossref] [PubMed]
- Doebele RC, Drilon A, Paz-Ares L, et al. Entrectinib in patients with advanced or metastatic NTRK fusion-positive solid tumours: integrated analysis of three phase 1–2 trials. Lancet Oncol 2020;21:271-82. [Crossref] [PubMed]
- Le DT, Uram JN, Wang H, et al. PD-1 Blockade in Tumors with Mismatch-Repair Deficiency. N Engl J Med 2015;372:2509-20. [Crossref] [PubMed]
- Marquart J, Chen EY, Prasad V. Estimation of the Percentage of US Patients With Cancer Who Benefit From Genome-Driven Oncology. JAMA Oncol 2018;4:1093-8. [Crossref] [PubMed]
- Shaw AT, Kim DW, Nakagawa K, et al. Crizotinib versus chemotherapy in advanced ALK-positive lung cancer. N Engl J Med 2013;368:2385-94. [Crossref] [PubMed]
- Galot R, Le Tourneau C, Guigay J, et al. Personalized biomarker-based treatment strategy for patients with squamous cell carcinoma of the head and neck: EORTC position and approach. Ann Oncol 2018;29:2313-27. [Crossref] [PubMed]
- Le Tourneau C, Kamal M, Alt M, et al. The spectrum of clinical trials aiming at personalizing medicine. Chin Clin Oncol 2014;3:13. [PubMed]
- von Hoff DD, Stephenson JJ, Rosen P, et al. Pilot study using molecular profiling of patients' tumors to find potential targets and select treatments for their refractory cancers. J Clin Oncol 2010;28:4877-83. [Crossref] [PubMed]
- Le Tourneau C, Delord JP, Gonçalves A, et al. Molecularly targeted therapy based on tumour molecular profiling versus conventional therapy for advanced cancer (SHIVA): a multicentre, open-label, proof-of-concept, randomised, controlled phase 2 trial. Lancet Oncol 2015;16:1324-34. [Crossref] [PubMed]
- Massard C, Michiels S, Ferte C, et al. High-Throughput Genomics and Clinical Outcome in Hard-to-Treat Advanced Cancers: Results of the MOSCATO 01 Trial. Cancer Discov 2017;7:586-95. [Crossref] [PubMed]
- Rodon J, Soria JC, Berger R, et al. Genomic and transcriptomic profiling expands precision cancer medicine: the WINTHER trial. Nat Med 2019;25:751-8. [Crossref] [PubMed]
- Chen AP, Kummar S, Moore N, et al. Molecular Profiling-Based Assignment of Cancer Therapy (NCI-MPACT): A Randomized Multicenter Phase II Trial. JCO Precis Oncol 2021;5:133-44. [Crossref] [PubMed]
- Flaherty KT, Gray RJ, Chen AP, et al. Molecular Landscape and Actionable Alterations in a Genomically Guided Cancer Clinical Trial: National Cancer Institute Molecular Analysis for Therapy Choice (NCI-MATCH). J Clin Oncol 2020;38:3883-94. [Crossref] [PubMed]
- Le Tourneau C, Kamal M, Tsimberidou AM, et al. Treatment Algorithms Based on Tumor Molecular Profiling: The Essence of Precision Medicine Trials. J Natl Cancer Inst 2015;108:djv362 [Crossref] [PubMed]
- Available online: https://www.curematch.com
- Stransky N, Cerami E, Schalm S, et al. The landscape of kinase fusions in cancer. Nat Commun 2014;5:4846. [Crossref] [PubMed]
- Belin L, Kamal M, Mauborgne C, et al. Randomized phase II trial comparing molecularly targeted therapy based on tumor molecular profiling versus conventional therapy in patients with refractory cancer: cross-over analysis from the SHIVA trial. Ann Oncol 2017;28:590-6. [Crossref] [PubMed]
- Dickson D, Johnson J, Bergan R, et al. The Master Observational Trial: A New Class of Master Protocol to Advance Precision Medicine. Cell 2020;180:9-14. [Crossref] [PubMed]
- Booth CM, Karim S, Mackillop WJ. Real-world data: towards achieving the achievable in cancer care. Nat Rev Clin Oncol 2019;16:312-25. [Crossref] [PubMed]
- EMA/763513/2018 Discussion paper: Use of patient disease registries for regulatory purposes – methodological and operational considerations. Available online: https://www.ema.europa.eu
. Available online: https://www.fda.orgFramework for FDA's Real-World Evidence Program