The B7-H1/PD-1 pathway in cancers associated with infections and inflammation: opportunities for therapeutic intervention
Epidemiology of cancers associated with inflammation
Approximately 16% of all malignancies world-wide are linked to viral and bacterial infections, which cause chronic inflammation (1,2). More than 90% of these cancers are attributed to 4 infectious organisms: Hepatitis viruses (B and C), high-risk type human papilloma viruses, and helicobacter pylori, which are linked to hepatocellular cancer, gastric cancer, and squamous cell cancers of the oropharynx, cervix, and other origins, respectively. Other infectious organisms etiologically linked to cancers comprise Epstein Barr virus (Hodgkin’s lymphoma, Burkitt’s lymphoma, and nasopharyngeal carcinoma), Human Herpes Virus type 8 (HHV-8), Human T-cell lymphotropic virus type 1 (HLTV-1) (Adult T-cell leukemia and lymphoma), liver flukes (cholangiocarcinoma), and schistosoma hematobium (bladder carcinoma). There are large geographical differences in the overall percentages of malignancies attributable to infection, reaching from 3.3% in Australia and New Zealand to 26.1% in China and 32.7% in Sub-Saharan Africa. Furthermore, there are differences in the relative contribution of infectious organisms to cancer burden; for example a higher proportion of gastric cancers are linked to H. pylori in more developed compared to less developed regions, whereas the reverse is true for hepatocellular cancers and Hepatitis B/C infection.
Inflammation and the tumor microenvironment
In addition to direct host-virus interactions such as viral integration into the host genome and targeted activation of oncogenic pathways by viral proteins, the mechanisms of tumorigenesis caused by infectious organisms are largely inflammatory (3,4). Various types of immune cells including macrophages, dendritic cells (DC), T cells, neutrophils, mast cells, myeloid derived suppressor cells (MDSC), tissue macrophages, and tumor associated macrophages (TAM) migrate to the tumor microenvironment. These cells are attracted by chemokines and directed by interactions with stromal/tumor cells through surface receptors such as adhesion molecules (e.g., members of the selectin family L-, P-and and E-Selectin) (3). Integrins on leukocyte membranes interacting with endothelial cells through vascular cell adhesion molecule-1 (VCAM-1) orchestrate the directed invasion of leukocytes into the tumor microenvironment. Furthermore, immune cells as well as tumor and stromal cells produce a multitude of cytokines (e.g., IL-6, IL-8, IL-10, VEGF, TNF-α, TGF-beta), angiogenic and other soluble factors, promoting tumorigenesis through multiple autocrine and paracrine loops. Extracellular proteases such as matrix metalloproteinases are also involved in facilitating the migration of various cells through the endothelium to the site of inflammation. These mechanisms suggest that the innate immune response triggered by infectious agents is intimately linked to cancer initiation and progression.
The adaptive immune response to tumor cells is intricately connected with the innate response and the inflammatory process. These responses are generally viewed as directed at tumor surveillance and prevention/eradication of tumor cells through recognition of tumor (neo) antigens as non-self. Nonetheless, even T cells specific for viral antigens such as the HBV surface antigen and B cells have been implicated in the propagation of inflammation and tumor progression (4-6). Cells of the innate immune system are an integral part of the priming and effector phases of T cell responses; for instance, the presentation of antigen to T cells by macrophages and DCs is critical for the T cell response. The interplay of both adaptive and innate immune responses in the inflammatory tumor environment, driving both cancer development and progression as well as immune-mediated tumor rejection is highly complex. In tumors that become established, the net impact favors tumor promotion (Figure 1).
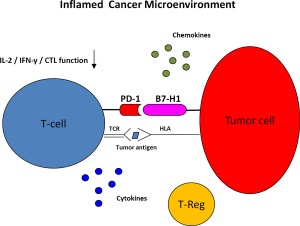
Targeting immune checkpoint pathways upregulated in the inflammatory tumor environment
A potential means to combat neoplasms that arise in the context of infection and chronic inflammation could involve immune checkpoint pathways, based on the known biology of host immune responses. Specifically, PD-1 is an inhibitory receptor expressed on the surface of activated T cells. PD-1 is a known marker of T-cell exhaustion in animal models of viral infection. This manifests itself as loss of effector functions such as the secretion of cytokines (IFN-γ, IL-2, TNF-α), production of the cytolytic effector molecules perforin and granzyme B, and eventually, apoptosis (7-9). The immune infiltrates of chronic inflammation frequently employ the B7-H1/PD-1 axis. Both PD-1 ligands, B7-H1 (PD-L1) and B7-DC (PD-L2) are up-regulated in peripheral tissues during an inflammatory response to infectious agents, in response to type I (α, β) and type 2 (IFN-γ) interferons (10). The biologic role of this upregulation is the prevention of collateral tissue damage mediated by antigen-experienced T cells during inflammation (11-14). Other immune-checkpoint molecules such as 2B4, CD160, T cell Immunoglobulin and Mucin domain-3 (Tim-3), Lymphocyte Activation Gene-3 (Lag-3) are upregulated in conjunction with PD-1 on “exhausted” CD8 T cells in tumor and chronic viral models.
Tumor activity of B7-H1/PD-1 pathway blockade in patients with advanced solid tumors
Tumor cells of various origins have been found to upregulate B7-H1 expression. Frequently, tumor infiltrating lymphocytes express PD-1 on their surface (15-19). Therapy with monoclonal antibodies directed against PD-1 and B7-H1 have recently shown encouraging successes in advanced melanoma, RCC, and metastatic non-small cell lung cancer (NSCLC). Objective tumor responses were seen in 18% of heavily pre-treated patients with advanced non-small cell lung cancer, 27% of patients with RCC, and 28% of patients with advanced melanoma treated in a phase I study with the IgG4 monoclonal antibody MDX-1106 (BMS, Bristol-Myers-Squibb) (20). More than two thirds of the responses lasted for more than one year. The treatment was well tolerated with grade 3 or 4 adverse events in the range of 15% and consisting predominantly of likely immune-related events affecting skin, lung, liver, gastrointestinal, and endocrine systems (20). Interestingly, none of 17 patients whose tumors were negative for B7-H1 had a response, whereas 9 of 25 patients (36%) with B7-H1 positive tumors experienced an objective response, suggesting that tumor B7-H1 expression may be a predictive marker for treatment with PD-1 blockade.
A monoclonal antibody directed against B7-H1 (MDX-1105) (BMS) induced objective tumor responses in 5 of 49 patients (10%) with extensively pre-treated metastatic non-small cell lung cancer, in 2 of 17 patients (12%) with RCC, and in 9 of 52 patients (17%) with melanoma (21). Treatment with this antibody was also well tolerated with grade 3 or 4 adverse events observed in less than 10% of patients.
The inflammatory context of cancers linked to viral or bacterial infection and associated overexpression of B7-H1 suggests that these tumors may be ideal candidates for therapy with immune checkpoint blockade. As will be discussed in the following sections, B7-H1 is overexpressed in many tumors associated with infections and chronic inflammation, likely mediated by type I (α, β) and type 2 (IFN-γ) interferons. Furthermore, T cells infiltrating these tumors express PD-1. It is intriguing that in cancers associated with infections and chronic inflammation, the B7-H1/PD-1 axis is upregulated not only as a result of a tumor immune defense mechanism, but also naturally in response to the inflammation mediated by the infectious organism. The inflammatory context leading to upregulation of the B7-H1/PD-1 pathway in these tumors, in conjunction with the demonstrated anti-tumor activity of PD-1 and B7-H1 blockade in melanoma, RCC, and NSCLC therefore suggests that B7-H1/PD-1 inhibition may be particularly valuable in these tumors.
The preclinical and clinical role of the B7-H1/PD-1 pathway in cancers associated with infections and chronic inflammation
Hepatocellular cancer (HCC)
PD-1 expression on peripheral T cells and B7-H1 expression on peripheral mononocytes was correlated with tumor progression in 141 patients with Hepatitis B-related HCC (22). Intratumoral and peripheral B7-H1 expression was positively correlated in the 23 patients for whom tumor tissue was available (22). Furthermore, expression of B7-H1 in tumor tissue from patients with HCC was associated with tumor vascular invasion as well as shorter disease-free and overall survival (23). An intriguing observation is the association of de novo hepatocarcinogenesis as defined by late (>1 year) intrahepatic recurrence with increased B7-H1 expression (23). Multicentric HCC occurs in 20-60% of patients with HCC after resection and is thought to be mediated by continuous viral infection and chronic inflammation, paralleling the pathogenesis of the primary HCC (24). PD-1 expression has been associated with Hepatitis B and C-specific T cell dysfunction, which is reversible by anti-B7-H1 blockade (25-29). The data suggest that B7-H1/PD-1 pathway blockade may be beneficial in the context of primary Hepatitis B/C associated HCC prevention, for the treatment/prevention of intra-hepatic second primary HCC, and for the treatment of metastatic HCC. Of particular interest with regard to chronic hepatitis C and hepatitis C-mediated HCC is the upregulation of B7-H1 by interferon-α, a type 2 interferon, which is part of any standard treatment regimen for Hepatitis C. This potential synergy provides a strong rationale for PD-1 blockade in this patient population; a phase 1 clinical trial assessing the safety and tumor activity of the anti-PD1 antibody MDX-1106 in patients with advanced HCC is currently ongoing (30).
Gastric cancer
Gastric cancer is one of the leading causes of cancer mortality worldwide (24). Helicobacter Pylori (H. Pylori) infection of the gastric mucosa leads to chronic gastritis and gastric/duodenal ulcers. Infection of the gastric mucosa with H. Pylori induces infiltration with T and B cells, macrophages, and neutrophils (31,32). Gastric epithelial cells lines upregulate B7-H1 upon exposure to H. pylori and gastric mucosa from H. Pylori infected subjects is higher compared to H. pylori negative subjects (33). Moreover, proliferation and IL-2 secretion of T cells co-cultured with H. Pylori infected gastric epithelial cells were found to be downregulated, suggesting a potential role in the adaptive immune response directed at premalignant or malignant cells during gastric carcinogenesis (33). H. Pylori is a critical element in the carcinogenesis of gastric cancer as demonstrated by the presence of the organism in gastric cancer samples and premalignant lesions of gastric mucosa (34), the strong association between H. Pylori Infection and gastric cancer shown in many studies (35), and the ability of the H. Pylori to cause gastric cancer in animal models (36). Evidence from two small studies indicates that B7-H1 and PD-1 may be upregulated in human gastric carcinoma samples as assessed by immunohistochemistry and RT-PCR (37,38). However, the role of B7-H1 in the gastric tumor immune-microenvironment has not been studied extensively to date. Of note, seven patients with advanced gastric cancer were included in the phase 1 study of the B7-H1 directed monoclonal antibody MDX-1105 (BMS) (21), whereas no patients with gastric cancer were enrolled in the phase 1 study of the PD-1 antibody MDX-1106 (BMS). No objective responses were seen in the 7 gastric cancer patients treated with MDX-1105. Nevertheless, given the importance of the B7-H1/PD-1 axis in the inflammatory context and the inflammatory pathogenesis of gastric carcinoma in conjunction with the lack of effective systemic therapies in the advanced stage, more comprehensive investigation of the B7-H1/PD-1 pathway or other immune checkpoint molecules such as Tim-3 or LAG-3 should be a potentially fruitful undertaking in gastric cancer.
Squamous cell carcinoma of the oropharynx
Squamous cell cancers of the head and neck are diagnosed in approximately 500,000 individuals worldwide each year (39). In contrast to the traditional risk factors for head and neck cancers, smoking and alcohol consumption, up to 80% of oropharyngeal cancers (particularly of the tonsils and the base of the tongue) are now associated with Human Papillomavirus subtype 16 (HPV-16) and carry a much better prognosis than HPV-negative tumors (40,41). HPV-16 infected cancer cells express the virally derived oncoproteins E6 and E7; T cells specific for E7 have been detected with increased frequencies in the peripheral blood of patients with HPV E7 positive tumors compared to patients with HPV E7 negative tumors and were found to be reactive in vitro against E7 positive tumor cells (42,43). The majority of HPV positive tumors are infiltrated with T cells and exhibit a gene signature consistent with an adaptive immune response, suggesting that HPV infection may induce a tumor-specific T cell response (44,45). The induction of an immune response may be one explanation for the improved outcome in this subset of squamous cell cancers. Nevertheless, tumor progression despite the induction of an immune response suggests the presence of immune evasion, presumably including the upregulation of immune checkpoint molecules on tumor cells, T-cells, or antigen presenting cells such as macrophages, dendritic cells, or B cells. There have been reports of PD-1 and B7-H1 overexpression in peripheral T cells and tumor samples from patients with oral squamous cell carcinoma (46-48), however, the data was generated in animal studies, cell lines, and small patient series. To our knowledge, patients with squamous cell carcinoma of the oropharynx have not been treated with PD-1 or B7-H1 inhibitors to date.
Nasopharyngeal cancer (NPC)
The incidence of NPC is 80,000 worldwide with 50,000 deaths every year (49). NPC is endemic in Southern China (especially in the Guandong and Guangxi provinces), Southeast Asia, North and Central Africa, the Middle East, and the Arctic (50). The etiology of NPC is complex and includes genetic, environmental, and viral components. Epstein-Barr Virus has been closely linked to NPC as is evident by the finding of EBV DNA in both precursor lesions and NPC tumor cells and the expression of EBV proteins such as EBNA-1, LMP1, and LMP2 (51-54). Although NPC is relatively radiotherapy and chemotherapy-sensitive, a significant proportion of patients develop locoregional or distant recurrence. NPC is categorized in 3 different histopathologic subtypes (WHO): (I) a well-differentiated, (II) moderately-differentiated, nonkeratinizing, and (III) undifferentiated. In southern China, the majority of tumors (>90%) are undifferentiated. A characteristic feature of NPC, particularly of the undifferentiated type, is the presence of a substantial immune infiltrate in the primary tumor consisting mainly of T cells, in addition to B cells, dendritic cells, monocytes, and eosinophils. Highly immunogenic antigens, such as the early lytic transactivator BZL1, have been identified in NPC tumor cells (55) and the antigen presenting function of NPC tumor cells as well as the function of peripheral EBV-specific T cells appear intact (56,57), suggesting that local immune suppression accounts for the inability of tumor specific T cells to clear malignant cells. There is evidence for functional inactivation of NPC primary tumor infiltrating lymphocytes (TIL): TIL from NPC, in contrast to peripheral T cells, failed to produce IFN-γ and to exert cytotoxicity when stimulated with autologous lymphoblastoid cell lines (LCL) (58). Recently, PD-1 expression on CD8 cells from NPC tumors was found to be upregulated compared to CD8 cells from control nasopharyngeal tissue (59). PD-1 overexpression was associated with shorter overall survival, disease-free survival, and recurrence-free survival and was an independent risk factor for death, treatment failure, and local recurrence of NPC, indicating that targeting the B7-H1/PD-1 pathway may benefit patients with NPC. Of note, no differences in B7-H1 expression were found between NPC tumor and control nasopharyngeal in that study. Other immunosuppressive mechanisms found in NPC tumors include immune checkpoint pathways such as Galectin-9/Tim-3 and CD4+CD25+FoxP3 regulatory cells (60,61).
EBV-associated lymphoma
Approximately half of the ca. 70,000 cases of Hodgkin’s lymphoma diagnosed world-wide every year are associated with EBV (2). EBV infection was recently shown to be linked to B7-H1 expression in primary Hodgkin’s lymphoma cells and EBV transformed lymphoblastoid cell lines with increased activity of both the B7-H1 promoter and enhancer. Furthermore, PD-1 was identified on EBV-specific CD8 cells in patients with acute infectious mononucleosis (62). These data support a role for B7-H1/PD-1 pathway inhibition in this group of lymphomas.
Concluding remarks
About one quarter of cancers diagnosed in China are inflammatory cancers that are linked to bacterial and viral pathogens. Approximately half of all hepatocellular carcinomas worldwide are diagnosed in China and more than 80% of them are associated with chronic Hepatitis B infection. Gastric cancer, which is related to H. Pylori infection, is the 3rd most common cancer worldwide in both men and women, while NPC is endemic in southern China. Inflammatory cancers, by their nature, occur in the context of an adaptive and innate immune response which is triggered initially by the infectious organism. The immune response then evolves into a complex interplay between T cells (specific to tumor antigens and/or infectious antigens), macrophages, monocytes, dendritic cells, and other cells of the innate immune response, stromal cells, in addition to soluble factors such as chemokines and cytokines. The balance of (anti-tumor and anti infectious organism-directed) adaptive T cell response and immune regulatory mechanisms as well as directly tumor promoting mechanisms in the tumor microenviroment of inflammatory tumors is most often tilted towards cancer progression. However, inflammation by itself is a positive prognostic marker in certain tumor types; for example in melanoma the inflamed phenotype seems to include the clinical responders to tumor vaccines (63). This may be explained by the fact that a tumor-specific T cell response is already present in the tumor environment, which, in a therapeutic sense “merely” needs to be uncoupled from the suppression by regulatory mechanisms. In this context, the identification of the B7-H1/PD-1 pathway as a critical immune regulatory checkpoint in conjunction with the recent demonstrated clinical efficacy of monoclonal antibodies targeting this pathway provides a strong rationale for B7-H1/PD-1 directed immunotherapy in cancers associated with infections and chronic inflammation.
Acknowledgements
Disclosure: The authors declare no conflict of interest.
References
- Parkin DM. The global health burden of infection-associated cancers in the year 2002. Int J Cancer 2006;118:3030-44.
- de Martel C, Ferlay J, Franceschi S, et al. Global burden of cancers attributable to infections in 2008: a review and synthetic analysis. Lancet Oncol 2012;13:607-15.
- Coussens LM, Werb Z. Inflammation and cancer. Nature 2002;420:860-7.
- Karin M, Greten FR. NF-kappaB: linking inflammation and immunity to cancer development and progression. Nat Rev Immunol 2005;5:749-59.
- Chisari FV. Hepatitis B virus transgenic mice: insights into the virus and the disease. Hepatology 1995;22:1316-25.
- de Visser KE, Korets LV, Coussens LM. De novo carcinogenesis promoted by chronic inflammation is B lymphocyte dependent. Cancer Cell 2005;7:411-23.
- Barber DL, Wherry EJ, Masopust D, et al. Restoring function in exhausted CD8 T cells during chronic viral infection. Nature 2006;439:682-7.
- Flies DB, Sandler BJ, Sznol M, et al. Blockade of the B7-H1/PD-1 pathway for cancer immunotherapy. Yale J Biol Med 2011;84:409-21.
- Wherry EJ. T cell exhaustion. Nat Immunol 2011;12:492-9.
- Mazanet MM, Hughes CC. B7-H1 is expressed by human endothelial cells and suppresses T cell cytokine synthesis. J Immunol 2002;169:3581-8.
- Carreno BM, Collins M. The B7 family of ligands and its receptors: new pathways for costimulation and inhibition of immune responses. Annu Rev Immunol 2002;20:29-53.
- Greenwald RJ, Freeman GJ, Sharpe AH. The B7 family revisited. Annu Rev Immunol 2005;23:515-48.
- Keir ME, Butte MJ, Freeman GJ, et al. PD-1 and its ligands in tolerance and immunity. Annu Rev Immunol 2008;26:677-704.
- Pentcheva-Hoang T, Corse E, Allison JP. Negative regulators of T-cell activation: potential targets for therapeutic intervention in cancer, autoimmune disease, and persistent infections. Immunol Rev 2009;229:67-87.
- Ahmadzadeh M, Johnson LA, Heemskerk B, et al. Tumor antigen-specific CD8 T cells infiltrating the tumor express high levels of PD-1 and are functionally impaired. Blood 2009;114:1537-44.
- Dong H, Strome SE, Salomao DR, et al. Tumor-associated B7-H1 promotes T-cell apoptosis: a potential mechanism of immune evasion. Nat Med 2002;8:793-800.
- Sfanos KS, Bruno TC, Meeker AK, et al. Human prostate-infiltrating CD8+ T lymphocytes are oligoclonal and PD-1+. Prostate 2009;69:1694-703.
- Topalian SL, Drake CG, Pardoll DM. Targeting the PD-1/B7-H1(PD-L1) pathway to activate anti-tumor immunity. Curr Opin Immunol 2012;24:207-12.
- Zou W, Chen L. Inhibitory B7-family molecules in the tumour microenvironment. Nat Rev Immunol 2008;8:467-77.
- Topalian SL, Hodi FS, Brahmer JR, et al. Safety, activity, and immune correlates of anti-PD-1 antibody in cancer. N Engl J Med 2012;366:2443-54.
- Brahmer JR, Tykodi SS, Chow LQ, et al. Safety and activity of anti-PD-L1 antibody in patients with advanced cancer. N Engl J Med 2012;366:2455-65.
- Zeng Z, Shi F, Zhou L, et al. Upregulation of circulating PD-L1/PD-1 is associated with poor post-cryoablation prognosis in patients with HBV-related hepatocellular carcinoma. PLoS One 2011;6:e23621.
- Gao Q, Wang XY, Qiu SJ, et al. Overexpression of PD-L1 significantly associates with tumor aggressiveness and postoperative recurrence in human hepatocellular carcinoma. Clin Cancer Res 2009;15:971-9.
- Llovet JM, Burroughs A, Bruix J. Hepatocellular carcinoma. Lancet 2003;362:1907-17.
- Boni C, Fisicaro P, Valdatta C, et al. Characterization of hepatitis B virus (HBV)-specific T-cell dysfunction in chronic HBV infection. J Virol 2007;81:4215-25.
- Peng G, Li S, Wu W, et al. PD-1 upregulation is associated with HBV-specific T cell dysfunction in chronic hepatitis B patients. Mol Immunol 2008;45:963-70.
- Mühlbauer M, Fleck M, Schütz C, et al. PD-L1 is induced in hepatocytes by viral infection and by interferon-alpha and -gamma and mediates T cell apoptosis. J Hepatol 2006;45:520-8.
- Chen C, Qu QX, Huang JA, et al. Expression of programmed-death receptor ligands 1 and 2 may contribute to the poor stimulatory potential of murine immature dendritic cells. Immunobiology 2007;212:159-65.
- Urbani S, Amadei B, Tola D, et al. PD-1 expression in acute hepatitis C virus (HCV) infection is associated with HCV-specific CD8 exhaustion. J Virol 2006;80:11398-403.
- NCT01658878 Cg. Multiple Ascending Dose (Anti-PD-1 HCC).
- D'Elios MM, Manghetti M, Almerigogna F, et al. Different cytokine profile and antigen-specificity repertoire in Helicobacter pylori-specific T cell clones from the antrum of chronic gastritis patients with or without peptic ulcer. Eur J Immunol 1997;27:1751-5.
- Di Tommaso A, Xiang Z, Bugnoli M, et al. Helicobacter pylori-specific CD4+ T-cell clones from peripheral blood and gastric biopsies. Infect Immun 1995;63:1102-6.
- Das S, Suarez G, Beswick EJ, et al. Expression of B7-H1 on gastric epithelial cells: its potential role in regulating T cells during Helicobacter pylori infection. J Immunol 2006;176:3000-9.
- Parsonnet J, Vandersteen D, Goates J, et al. Helicobacter pylori infection in intestinal- and diffuse-type gastric adenocarcinomas. J Natl Cancer Inst 1991;83:640-3.
- An international association between Helicobacter pylori infection and gastric cancer. The EUROGAST Study Group. Lancet 1993;341:1359-62.
- Watanabe T, Tada M, Nagai H, et al. Helicobacter pylori infection induces gastric cancer in mongolian gerbils. Gastroenterology 1998;115:642-8.
- Liu SM, Meng Q, Zhang QX, et al. Expression and significance of B7-H1 and its receptor PD-1 in human gastric carcinoma. Zhonghua Zhong Liu Za Zhi 2008;30:192-5.
- Lu B, Chen L, Liu L, et al. T-cell-mediated tumor immune surveillance and expression of B7 co-inhibitory molecules in cancers of the upper gastrointestinal tract. Immunol Res 2011;50:269-75.
- Jemal A, Bray F, Center MM, et al. Global cancer statistics. CA Cancer J Clin 2011;61:69-90.
- Chaturvedi AK, Engels EA, Pfeiffer RM, et al. Human papillomavirus and rising oropharyngeal cancer incidence in the United States. J Clin Oncol 2011;29:4294-301.
- Gillison ML, D’Souza G, Westra W, et al. Distinct risk factor profiles for human papillomavirus type 16-positive and human papillomavirus type 16-negative head and neck cancers. J Natl Cancer Inst 2008;100:407-20.
- Albers A, Abe K, Hunt J, et al. Antitumor activity of human papillomavirus type 16 E7-specific T cells against virally infected squamous cell carcinoma of the head and neck. Cancer Res 2005;65:11146-55.
- Hoffmann TK, Arsov C, Schirlau K, et al. T cells specific for HPV16 E7 epitopes in patients with squamous cell carcinoma of the oropharynx. Int J Cancer 2006;118:1984-91.
- Heusinkveld M, Goedemans R, Briet RJ, et al. Systemic and local human papillomavirus 16-specific T-cell immunity in patients with head and neck cancer. Int J Cancer 2012;131:E74-85.
- Thurlow JK, Peña Murillo CL, Hunter KD, et al. Spectral clustering of microarray data elucidates the roles of microenvironment remodeling and immune responses in survival of head and neck squamous cell carcinoma. J Clin Oncol 2010;28:2881-8.
- Chen J, Feng Y, Lu L, et al. Interferon-γ-induced PD-L1 surface expression on human oral squamous carcinoma via PKD2 signal pathway. Immunobiology 2012;217:385-93.
- Malaspina TS, Gasparoto TH, Costa MR, et al. Enhanced programmed death 1 (PD-1) and PD-1 ligand (PD-L1) expression in patients with actinic cheilitis and oral squamous cell carcinoma. Cancer Immunol Immunother 2011;60:965-74.
- Tsushima F, Tanaka K, Otsuki N, et al. Predominant expression of B7-H1 and its immunoregulatory roles in oral squamous cell carcinoma. Oral Oncol 2006;42:268-74.
- Ferlay J, Shin HR, Bray F, et al. Estimates of worldwide burden of cancer in 2008: GLOBOCAN 2008. Int J Cancer 2010;127:2893-917.
- Chang ET, Adami HO. The enigmatic epidemiology of nasopharyngeal carcinoma. Cancer Epidemiol Biomarkers Prev 2006;15:1765-77.
- Chien YC, Chen JY, Liu MY, et al. Serologic markers of Epstein-Barr virus infection and nasopharyngeal carcinoma in Taiwanese men. N Engl J Med 2001;345:1877-82.
- Pathmanathan R, Prasad U, Sadler R, et al. Clonal proliferations of cells infected with Epstein-Barr virus in preinvasive lesions related to nasopharyngeal carcinoma. N Engl J Med 1995;333:693-8.
- Heussinger N, Büttner M, Ott G, et al. Expression of the Epstein-Barr virus (EBV)-encoded latent membrane protein 2A (LMP2A) in EBV-associated nasopharyngeal carcinoma. J Pathol 2004;203:696-9.
- Khabir A, Karray H, Rodriguez S, et al. EBV latent membrane protein 1 abundance correlates with patient age but not with metastatic behavior in north African nasopharyngeal carcinomas. Virol J 2005;2:39.
- Cochet C, Martel-Renoir D, Grunewald V, et al. Expression of the Epstein-Barr virus immediate early gene, BZLF1, in nasopharyngeal carcinoma tumor cells. Virology 1993;197:358-65.
- Khanna R, Busson P, Burrows SR, et al. Molecular characterization of antigen-processing function in nasopharyngeal carcinoma (NPC): evidence for efficient presentation of Epstein-Barr virus cytotoxic T-cell epitopes by NPC cells. Cancer Res 1998;58:310-4.
- Lin X, Gudgeon NH, Hui EP, et al. CD4 and CD8 T cell responses to tumour-associated Epstein-Barr virus antigens in nasopharyngeal carcinoma patients. Cancer Immunol Immunother 2008;57:963-75.
- Li J, Zeng XH, Mo HY, et al. Functional inactivation of EBV-specific T-lymphocytes in nasopharyngeal carcinoma: implications for tumor immunotherapy. PLoS One 2007;2:e1122.
- Hsu MC, Hsiao JR, Chang KC, et al. Increase of programmed death-1-expressing intratumoral CD8 T cells predicts a poor prognosis for nasopharyngeal carcinoma. Mod Pathol 2010;23:1393-403.
- Klibi J, Niki T, Riedel A, et al. Blood diffusion and Th1-suppressive effects of galectin-9-containing exosomes released by Epstein-Barr virus-infected nasopharyngeal carcinoma cells. Blood 2009;113:1957-66.
- Lau KM, Cheng SH, Lo KW, et al. Increase in circulating Foxp3+CD4+CD25(high) regulatory T cells in nasopharyngeal carcinoma patients. Br J Cancer 2007;96:617-22.
- Greenough TC, Campellone SC, Brody R, et al. Programmed Death-1 expression on Epstein Barr virus specific CD8+ T cells varies by stage of infection, epitope specificity, and T-cell receptor usage. PLoS One 2010;5:e12926.
- Gajewski TF, Fuertes M, Spaapen R, et al. Molecular profiling to identify relevant immune resistance mechanisms in the tumor microenvironment. Curr Opin Immunol 2011;23:286-92.