Personalized radiation therapy for glioblastoma
Introduction
Glioblastoma is the most common primary central nervous system cancer with an estimated 14,000 Americans diagnosed with this disease every year (1). Postoperative radiation was first demonstrated to improve overall survival (OS) for patients with glioblastoma in 1978. A randomized trial enrolled 303 patients with anaplastic glioma who had undergone surgery to treatment with 1,3-bis(2-chloroethyl)-1-nitrosourea (BCNU) and/or radiation therapy, or observation (2) (Table 1). The results showed a doubling of OS in patients receiving radiation therapy, establishing radiation therapy as a standard of care treatment for patients with glioblastoma. These patients received 50–60 Gy to the whole brain. The discovery that most recurrences occurred in proximity to the tumor bed lead investigators to personalize radiation therapy by decreasing the radiation dose to the whole brain and boosting only the tumor bed to a higher dose in the Brain Tumor Cooperative Group Trial 8001 (3). This was a randomized trial testing different chemotherapy regimens. However, two different radiation volumes were used, allowing a comparison between whole brain radiation and more focal radiation as adjuvant treatment for glioblastoma. In the first cohort of patients, treatment was delivered with a dose of 60.2 Gy to the whole brain, while in a second cohort, patients were treated initially to 43 Gy to the whole brain followed by a cone down to the tumor volume to 17.2 Gy. There was no difference in outcome between the cohort of patients receiving whole brain radiation therapy (WBRT) and the group treated with the cone down, leading investigators to conclude that a coned-down boost was as effective as WBRT.
Table 1
Reference | Year | Patients, n | Inclusion criteria | Treatment arms | Survival outcome (median) |
---|---|---|---|---|---|
Walker MD (2) | 1978 | 303 | Anaplastic glioma | Surgery and then BCNU and RT (50-60 Gy to the whole brain), RT alone, BCNU alone, or supportive care | 34.5 weeks for BCNU + RT, 35.0 weeks for RT alone, 18.5 weeks for BCNU alone, 14.0 weeks for supportive care |
Shapiro WR (3) | 1989 | 571 | Anaplastic glioma (80% glioblastoma) | Surgery and then BCNU alone, BCNU and procarbazine, or BCNU and hydroxyurea and procarbazine. Radiation was either 60.2 Gy to the whole brain or 43 Gy to the whole brain with cone down to 60.2 Gy | 11.3 to 13.8 months, no significant difference between groups |
Stupp R (4) | 2005 | 573 | Glioblastoma | Surgery followed by RT to 60 Gy or RT to 60 Gy with TMZ | 12.1 months for RT vs. 14.6 months for RT and TMZ |
Stupp R (5) | 2017 | 695 | Glioblastoma | Surgery followed by RT to 60 Gy and TMZ vs. RT to 60 Gy, TMZ, and TTF | 16 months for RT and TMZ vs. 20.9 months for RT, TMZ, and TTF |
Wick W (6) | 2012 | 373 | Glioblastoma or anaplastic astrocytoma, age >65 years | Surgery followed by TMZ alone or RT to 60 Gy in 30 fx | 8.6 months for TMZ vs. 9.6 months for RT. EFS longer with MGMT methylation in the TMZ group (8.4 vs. 4.6 months). EFS longer with RT in the no MGMT methylation (4.6 vs. 3.3 months) |
Malmström A (7) | 2012 | 291 | Glioblastoma, age >60 years | TMZ alone, RT to 60 Gy in 30 fx, RT to 34 Gy in 10 fx | 8.3 months for TMZ, 7.5 months for 34 Gy in 10 fx, 6.0 months for 60 Gy in 30 fx |
Guo C (8) | 2023 | 199 | Glioblastoma or WHO grade 3 gliomas | Surgery and then 60 Gy in 30 fx with TMZ or 60 Gy in 30 fx, TMZ, and interferon alpha | 18.8 months for RT and TMZ vs. 26.7 months for RT, TMZ, and interferon alpha |
Weller M (9) | 2017 | 745 | Glioblastoma with EGFRvIII mutation | Surgery, then 60 Gy in 30 fx and TMZ vs. 60 Gy in 30 fx with TMZ and Rindopepimut | Amongst patients with minimal residual disease, 20.0 moths in control group and 20.1 months with Rindopepimut |
Souhami L (10) | 2004 | 203 | Glioblastoma ≤4 cm | Surgery and then SRS, 60 Gy in 30 fx, and BCNU vs. 60 Gy in 30 fx and BCNU | 13.5 months with SRS vs. 13.6 months without SRS |
Laprie A (11) | 2024 | 180 | Glioblastoma | Surgery then 60 Gy in 30 fx RT with TMZ vs. 60 Gy in 30 fx, TMZ, and boost to 72 Gy in 30 fx to MRSI sites likely for recurrence | 22.6 months in 60 Gy and TMZ arm vs. 22.2 months in 60 Gy, TMZ and MRSI boost to 72 Gy arm |
Brown PD (12) | 2021 | 90 | Glioblastoma | Surgery then 60 Gy in 30 fx with TMZ using photons vs. 60 Gy in 30 fx with TMZ using protons | 24.5 months for protons vs. 21.2 months for photons |
Roa W (13) | 2004 | 100 | Glioblastoma and age ≥60 years | Surgery then 60 Gy in 30 fx or 40 Gy in 15 fx | 5.1 months for 60 Gy vs. 5.6 months for 40 Gy |
Perry JR (14) | 2017 | 562 | Glioblastoma and age ≥65 years | Surgery then 40 Gy in 15 fx vs. 40 Gy in 15 fx with TMZ | 7.9 vs. 10.0 months |
BCNU, 1,3-bis(2-chloroethyl)-1-nitrosourea; RT, radiation therapy; TMZ, temozolomide; TTF, tumor treating fields; EFS, event-free survival; MGMT, O6-methylguanine-DNA methyltransferase; SRS, stereotactic radiosurgery; MRSI, magnetic resonance spectroscopic imaging.
Current management
The current treatment paradigm for patients with glioblastoma and a good performance status is maximal safe surgical resection followed by postoperative radiation to a total dose of 60 Gy in 30 fractions with concurrent chemotherapy and possibly use of tumor treatment fields (4,5,15). The superiority of chemoradiation compared to radiation alone was demonstrated by the Stupp trial first published in 2005. A total of 573 patients with glioblastoma received maximal safe resection and were subsequently randomized to receiving 60 Gy in 30 fractions or 60 Gy in 30 fractions along with concurrent and adjuvant temozolomide chemotherapy. At a median follow-up of 28 months, the median OS with chemoradiation was 14.6 months, compared to a median of 12.1 months with radiation alone, leading authors to conclude that the addition of chemotherapy to radiation was the new standard of care. One criticism of this trial is that the upper limit of age for patients enrolled was 71 years, and since the median age of diagnosis of patients with glioblastoma is 65 years, there is concern that these results may not be generalizable to a sizeable number of patients diagnosed with this disease. The next major breakthrough with respect to improving clinical outcomes for patients with glioblastoma came as a result of an antimitotic treatment called tumor treating fields (TTFields) (5). This is an antimitotic treatment device that delivers low-intensity intermediate frequency electric fields that causes mitotic arrest and apoptosis of rapidly dividing cells (16). In the seminal EF14 trial that first demonstrated the efficacy of TTFields (5), 695 patients with glioblastoma who had completed chemoradiation were randomized to standard chemotherapy alone or chemotherapy along with TTFields. The median OS was 20.9 months in the TTFields arm, and 16.0 months in the chemotherapy alone arm (P<0.001), leading authors to conclude that TTFields lead to improved OS in patients with glioblastoma.
Predictive biomarkers
The O6-methylguanine-DNA methyltransferase (MGMT) gene codes for a protein that removes alkyl products from the O6 position of guanine which is a site of DNA alkylation (17,18). The protein encoded by the MGMT gene must be replenished in order to continuing repairing DNA damage induced by alkylating agents, as without the DNA repair, cytotoxicity and apoptosis occur in cancer cells (19,20). Excessive activity of the MGMT encoded protein can repair the DNA damage in cancer cells induced by alkylating agents resulting in worse clinical outcomes (21,22).
Investigators hypothesized that inactivation of the DNA repair gene encoded by MGMT and first demonstrated this in a retrospective review of 47 patients with high grade glioma who has been treated with carmustine (23). In this study, Spanish investigators obtained the tumor specimens of 47 patients with high grade glioma who has been treated with surgery, radiation and chemotherapy and performed methylation-specific polymerase chain reaction (PCR) to determine whether or not patients had a methylated MGMT promoter, which would inactivate the DNA repair protein, and then correlate MGMT promoter methylation with outcome. The results demonstrated that MGMT promoter methylation was associated with improved progression-free survival (PFS) of 21 months for the methylated MGMT group compared to 8 months for the unmethylated group and improved OS [hazard ratio (HR) for death of 10.8 for patients without MGMT methylation]. This study was criticized due to the heterogeneity of the patient population, which lead investigators to further explore the relationship between MGMT methylation and clinical outcomes for patients with glioblastoma.
The first randomized data to demonstrate the predictive value of MGMT methylation for patients with glioblastoma was published in 2004. This data was obtained from a phase II trial testing the efficacy of radiation along with temozolomide based chemotherapy, researchers explored outcomes for 49 patients with glioblastoma treated with surgery, chemotherapy, and radiation after stratifying patients by MGMT promoter methylation status (18). At 18 months, 62% of patients with MGMT promoter methylation were alive, compared to 8% of patients without MGMT promoter methylation, leading to the conclusion that MGMT-methylation status was an independent predictor for patients with glioblastoma treated with alkylating chemotherapy. Investigators from the aforementioned Stupp trial tested the pathology specimens of patients enrolled in the trial to determine whether MGMT promoter methylation for patients with glioblastoma was associated with benefit with temozolomide treatment (24). MGMT promoter methylation was tested on 206 out of the 573 patients enrolled in the trial and outcomes were compared in each treatment arm after stratifying for patients with MGMT methylation status. Improved median OS was observed when comparing patients with MGMT promoter methylation treated with chemotherapy and radiation (21.7 months for the chemoradiation arm compared to 15.3 months with radiation alone). However, when comparing outcomes for patients without MGMT methylation, there was no statistically significant improvement in OS when comparing the two patient cohorts (median OS 12.7 months with chemoradiation compared to 11.8 months with radiation alone, P=0.06). These results lead the investigators to conclude that MGMT promoter methylation is a clinically relevant predictor of the use of temozolomide chemotherapy, and that additional treatments should be developed to improve outcomes amongst patients without the MGMT promoter methylation.
MGMT promoter methylation can be used to personalize treatment for elderly patients with glioblastoma. In the NOA-08 trial, investigators sought to determine whether chemotherapy could replace radiation therapy as definitive adjuvant treatment for elderly patients (defined as age >65 years) with glioblastoma or anaplastic astrocytoma (6). In this multi-institutional, phase III trial, 373 patients with glioblastoma age >65 years were treated with surgery and subsequently randomized to either temozolomide based chemotherapy alone or radiation therapy alone to a dose of 60 Gy in 30 fractions. OS and event-free survival (EFS) were similar in both treatment groups. On subset analysis, amongst patients with MGMT promoter methylation, EFS was longer in patients receiving temozolomide compared to those receiving radiation therapy (8.4 vs. 4.6 months). Amongst patients without MGMT promoter methylation, EFS was longer in patients receiving radiation therapy compared to those receiving temozolomide (4.6 vs. 3.3 months). The Nordic III trial also sought to determine the optimal treatment for elderly patients (7). A total of 291 patients with glioblastoma and age >60 years were randomized to receive either temozolomide alone, hypofractionated radiation therapy (HFRT) defined here as 34 Gy in 10 fractions, or conventionally fractionated radiation therapy (CFRT) defined as 60 Gy in 30 fractions. OS was significantly greater in patients receiving temozolomide alone (8.3 months) than in patients receiving CFRT alone (6.0 months, P=0.01), though not greater than patients receiving HFRT (7.5 months, P=0.24). Amongst patients treated with temozolomide, a greater median OS was observed amongst patients with MGMT promoter methylation than in patients without the MGMT promoter methylation. However, amongst patients treated with radiation, no difference in OS was noted between patients with or without MGMT promoter methylation. As a result of these trials, the current National Comprehensive Cancer Network (NCCN) Guidelines for management of elderly patients with glioblastoma allows treatment with chemotherapy alone as an option for patients with a methylated MGMT promoter, though not for patients with an unmethylated MGMT promoter region.
Patients without MGMT promoter methylation have been demonstrated to have worse clinical outcomes with the use of alkylating chemotherapy. Researchers having been conducting experiments to overcome the relative resistance to alkylating chemotherapy amongst patients without the MGMT promoter methylation. One such therapeutic intervention is the use of interferon alpha, which is associated with the innate immune system and has both immunomodulatory, antiproliferative, and antiangiogenic properties (25). Importantly, Chinese investigators demonstrated in 2015 that interferon alpha enhances the efficacy of temozolomide by downregulating MGMT expression (26). Subsequently, Guo et al. conducted a Phase III randomized trial in patients with high grade glioma to test the efficacy of interferon alpha in patients with high grade glioma treated with surgery, radiation, and chemotherapy (8). In this multi-center trial, 199 patients with glioblastoma and WHO grade 3 gliomas were randomized after surgery to receive treatment with CFRT and temozolomide or radiation therapy, temozolomide, along with interferon alpha. The median OS was greater in patients receiving interferon alpha (26.7 vs. 18.8 months, P=0.005). On subset analysis, amongst patients without MGMT methylation, use of interferon alpha was associated with improved median OS (24.7 vs. 17.4 months). The finding that interferon alpha can significantly improve clinical outcomes for patients without MGMT methylation is significant in that these patients otherwise have been shown to have a worse prognosis.
Mutation of the epidermal growth factor receptor (EGFR) is found in about 50% of malignant gliomas (27). EGFR dysregulation can lead to increased tumor angiogenesis, migration, growth, and spread (28), and is associated with worse clinical outcomes in patients with glioblastoma (29). It has been hypothesized that targeting EGFR mutation may lead to improved outcomes for patients with glioblastoma. Unfortunately, randomized trials have shown no benefit with the addition of EGFR targeted therapy for patients with glioblastoma (9-31). In the largest phase III trial testing out the potential efficacy of EGFR targeted therapy (9), 745 patients with glioblastoma confirmed to express EGFRvIII were treated with surgery and standard chemoradiation after which they were randomized to either Rindopepimut, a vaccine targeting the EGFR deletion mutation EGFRvIII, or placebo. No differences in OS were observed with the use of Rindopepimut, leading investigators to conclude that future work was needed to determine if EGFR targeted therapy could be of benefit in patients with glioblastoma.
Radiation dose and technique
Intensity modulated radiation therapy (IMRT) is a radiation technique that uses inverse planning algorithms to deliver a high radiation dose to tumor while sparing dose from adjacent structures (32). The use of multiple radiation beams from different angles allows for higher dose to the gross disease with simultaneous sparing of healthy brain and radiation sensitive structures, which has been demonstrated to reduce toxicity associated with radiation (33,34). Volumetric modulated arc therapy (VMAT) is an advanced radiation technique that modulates dose rate, field aperture, and gantry speed during the delivery of radiation which creates a more conformal dose distribution, allowing more sparing of normal tissues than compared with IMRT treatment plans (35,36). However, while the dosimetric improvements of VMAT when compared to IMRT have not translated to improved clinical outcomes or decreased toxicity, VMAT does result in a mean 29% reduction in treatment time, and should be considered as a treatment technique for patients with glioblastoma (37).
The standard radiation dose used to treat glioblastoma is 60 Gy in 30 fractions. The poor prognostic associated with this disease has led investigators to attempt to deliver dose-escalated radiation therapy (DE-RT) in an effort to improve clinical outcomes for patients. In one such phase III randomized trial, researchers sought to determine whether a stereotactic radiosurgery (SRS) boost could improve OS for patients with glioblastoma (10). Two hundred and three patients with glioblastoma measuring ≤40 mm were treated with surgery and then randomized to treatment with CFRT, BCNU, and SRS to a dose of 15 to 24 Gy (SRS dose determined by size) or CFRT and BCNU alone. At a median follow-up time of 61 months, there was no difference in median survival in the two patient cohorts (13.5 vs. 13.6 months), leading investigators to conclude that there was no benefit with the SRS boost.
A meta-analysis recently explored outcomes for DE-RT for patients with glioblastoma (38). A total of 22 studies were examined, in which 2198 patients with glioblastoma received definitive treatment, out of which 507 received DE-RT. When comparing outcomes between patients treated with adjuvant radiation alone, 1-year OS was improved with the use of DE-RT (46.3% vs. 23.4%, P=0.02). However, no significant difference in 1-year OS was observed when compared to patients who received chemotherapy along with radiation between the DE-RT and standard radiation therapy cohorts (73.2% vs. 64.5%, P=0.23). Similarly, no difference in outcomes between DE-RT and standard radiation therapy when used concurrently with chemotherapy was observed amongst patients with MGMT methylation (1-year OS 83.2% vs. 73.2%, P=0.23) or without MGMT methylation (1-year OS 72.6% vs. 50.6%, P=0.16). Laprie et al. attempted using magnetic resonance spectroscopic imaging (MRSI) to identify sites most likely for recurrence and to use DE-RT to selectively boost these regions (11). One hundred and eighty patients with glioblastoma were treated with surgery and randomized to chemotherapy and either a standard radiation dose of 60 Gy in 30 fractions or the standard radiation dose with an additional simultaneous integrated boost to 72 Gy to MRSI metabolic abnormalities, the tumor bed, and residual contrast enhancement. At a median follow-up time of 43.9 months, the median OS was 22.6 months in the standard radiation dose arm compared to 22.2 months in the DE-RT arm. The investigators concluded that there was no improvement in clinical outcome with MRSI guided DE-RT.
Proton based radiation has been suggested to be a possible radiation technique to either decrease cognitive deficit or to improve outcomes for patients with glioblastoma. In a phase II trial, 90 patients with glioblastoma were treated with surgery and then randomized to treatment with radiation therapy to a dose of 60 Gy in 30 fractions with concurrent temozolomide using either proton therapy or photon based radiation (12). At a median follow-up time of 48.7 months, there was no significant difference in either time to cognitive failure (HR =0.88, P=0.74) or OS (HR =0.68, P=0.60). A multicenter prospective registry study from proton beam facilities in Japan reported a median OS for patients with glioblastoma treated with proton based radiation therapy to be 21.2 months, which investigators concluded was equivalent to reported treatment outcomes for patients with glioblastoma (39). A meta-analysis of trials in which patients with glioblastoma were treated with proton based radiation therapy also demonstrated clinical outcomes similar to those found in patients treated with photon based radiation (40). These results suggest that proton based radiation may not significantly improve clinical outcomes for patients with glioblastoma.
Radiation volumes
Despite consensus on the standard postoperative radiation dose in otherwise healthy patients, there is considerable variation in the recommended radiation volume to be treated. The European Organisation for Research and Treatment of Cancer (EORTC) has recently published contouring guidelines when treating glioblastoma (41), that recommends treating the gross residual disease as identified on magnetic resonance imaging (MRI) T1 contrast enhancing tumor and/or the resection cavity plus an additional 15 mm margin to create a clinical target volume (CTV). The T2/fluid attenuated inversion recovery abnormality (FLAIR) signal abnormality is recommended to be included as part of the CTV, but inclusion of edema is not recommended. The dose is recommended to be delivered to a single volume with no cone down of treatment volume recommended (41). However, these guidelines are not universally followed. The NRG Oncology Group has its own set of guidelines, which recommend treating an initial CTV as delineated by the T2/FLAIR sequence as seen on postoperative MRI plus a 2 cm expansion to 46 Gy in 23 fractions followed by a boost to the T1/post contrast plus a 2 cm expansion to an additional 14 Gy in 7 fractions (42) (Figure 1). Other institutions have used 0.5 to 1 cm CTV margins added to the T1 postcontrast sequence and demonstrated only 5–10% out of field failure (Figure 2) (43,44), suggesting that decreased margin size may be reasonable and that smaller treatment margins may also lead to decreased risk of lymphopenia (45).
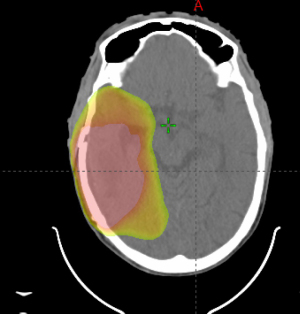
Special considerations
Multifocal glioblastoma has been shown to be associated with worse OS than unifocal glioblastoma, likely due to the biological aggressiveness of disease (46) (Figure 2). Superior OS in patients with multifocal disease is observed following gross total resection as well as concurrent chemotherapy and radiation therapy to a dose of 60 Gy in 30 fractions (47).
Older patients with glioblastoma and a poor performance status have been found to have worse prognosis, with limited long-term survival (48). Consequently, investigators sought to determine whether radiation therapy could be personalized in older patients in order to minimize the logistical challenge associated with a six-week course of radiation without compromising clinical outcome. In 2004, Roa et al. randomized 100 patients with glioblastoma aged 60 years or greater to maximal safe resection followed by either CFRT to 60 Gy in 30 fractions or HFRT to a dose of 40 Gy in 15 fractions (13). The results showed no difference in OS with the HFRT course of treatment, leading investigators to conclude that the HFRT course of treatment may be preferable for elderly patients. The initial trial demonstrating the clinical equipoise of HFRT to CFRT for elderly patients with glioblastoma was conducted prior to knowledge regarding the efficacy of concurrent chemoradiation using temozolomide. Researchers have since demonstrated that HFRT along with temozolomide leads to improved OS when compared to HFRT alone for elderly patients, leading the authors to conclude that HFRT and chemotherapy should be the standard treatment for elderly patients who could tolerate dual modality adjuvant treatment (14,49).
Future directions
The current median OS for patients with glioblastoma is 15–20 months. More effective treatments are needed to improve outcomes for these patients. The NRG BN-001 trial is a phase II randomized trial enrolling patients following surgical resection to either standard-dose radiation to a dose of 60 Gy in 30 fractions or dose escalated radiation to a dose of 75 Gy in 30 fractions along with concurrent chemotherapy using either photon-based or proton-based radiation (50). The initial results in the photon-based dose escalation arm did not show improved outcomes when compared to the standard-dose radiation. The proton-based cohort has now completed accrual, with results yet to be released.
The Trident trial is attempting to improve upon the OS improvements demonstrated in the aforementioned EF-17 trial with the use of TTFields (6,51). This is an international phase III trial that is randomizing patients to upfront surgical resection followed by adjuvant standard chemoradiation and subsequent chemotherapy and TTFields, or surgery followed by chemoradiation with concurrent use of TTfields, with subsequently chemotherapy and TTFields.
Investigators are exploring using a Bayesian machine-learning framework to create personalized radiation therapy plans that use information from MRI scans and fluoro-ethyl-tyrosine (FET)-positron emission tomography (PET) metabolic maps to determine tumor cell density in glioblastoma patients (52). This information is used to create personalized radiation plans taking into account tumor cell density and a clinical population study has demonstrated that the radiation therapy plans created using this method can reduce radiation toxicity when compared to conventional plans. However, this technique has not yet been tested in phase III randomized trials, though is a promising method to allow for greater radiation dose delivery to sites where tumors are most likely to recur. A second method being investigated to create personalized radiation therapy plans is using deep learning based free water correction of diffusion tensor imaging to determine the infiltration of tumor within the FLAIR region of MRI scans (53). Investigators are trying to use the free water corrected diffusion-tensor imaging (DTI) images to create personalized radiation therapy plans that may potentially escalate radiation dose in sites with greater tumor infiltration where cancers are most likely to recur, while possibly allowing for dose de-escalation in regions with less tumor infiltration. Resveratrol has been suggested as a compound that could be used in conjunction with radiation therapy due to its potential benefits as a radiation sensitizer and chemotherapy sensitizer, though this has yet to be demonstrated in clinical practice (54). Use of high Z nanoparticles has also been suggested as a method to enhance the effectiveness of radiation therapy when treating glioblastoma and has shown efficacy in preclinical models, though clinical studies demonstrating its feasibility and efficacy have yet to be conducted (55).
Conclusions
Current management of glioblastoma includes maximal safe surgical resection after which management is contingent upon the patient’s age, performance status, and MGMT methylation status. While young patients with a good performance status are treated with postoperative radiation to the tumor bed and any residual gross disease to a dose of 60 Gy in 30 fractions with concurrent and adjuvant temozolomide along with TTFields, elderly patients may receive HFRT alone or temozolomide alone in cases in which the tumor is MGMT methylated. Future studies are needed to improve outcomes in all patients, particularly in the MGMT unmethylated patient population. The use of interferon alpha is promising as an addition to the standard of care, and future studies will confirm the benefit of this chemotherapeutic agent, which has the potential of increased benefit in the MGMT unmethylated patient population.
Acknowledgments
Funding: None.
Footnote
Peer Review File: Available at https://cco.amegroups.com/article/view/10.21037/cco-23-107/prf
Conflicts of Interest: All authors have completed the ICMJE uniform disclosure form (available at https://cco.amegroups.com/article/view/10.21037/cco-23-107/coif). The authors have no conflicts of interest to declare.
Ethical Statement:
Open Access Statement: This is an Open Access article distributed in accordance with the Creative Commons Attribution-NonCommercial-NoDerivs 4.0 International License (CC BY-NC-ND 4.0), which permits the non-commercial replication and distribution of the article with the strict proviso that no changes or edits are made and the original work is properly cited (including links to both the formal publication through the relevant DOI and the license). See: https://creativecommons.org/licenses/by-nc-nd/4.0/.
References
- Miller KD, Ostrom QT, Kruchko C, et al. Brain and other central nervous system tumor statistics, 2021. CA Cancer J Clin 2021;71:381-406. [Crossref] [PubMed]
- Walker MD, Alexander E Jr, Hunt WE, et al. Evaluation of BCNU and/or radiotherapy in the treatment of anaplastic gliomas. A cooperative clinical trial. J Neurosurg 1978;49:333-43. [Crossref] [PubMed]
- Shapiro WR, Green SB, Burger PC, et al. Randomized trial of three chemotherapy regimens and two radiotherapy regimens and two radiotherapy regimens in postoperative treatment of malignant glioma. Brain Tumor Cooperative Group Trial 8001. J Neurosurg 1989;71:1-9. [Crossref] [PubMed]
- Stupp R, Mason WP, van den Bent MJ, et al. Radiotherapy plus concomitant and adjuvant temozolomide for glioblastoma. N Engl J Med 2005;352:987-96. [Crossref] [PubMed]
- Stupp R, Taillibert S, Kanner A, et al. Effect of Tumor-Treating Fields Plus Maintenance Temozolomide vs Maintenance Temozolomide Alone on Survival in Patients With Glioblastoma: A Randomized Clinical Trial. JAMA 2017;318:2306-16. [Crossref] [PubMed]
- Wick W, Platten M, Meisner C, et al. Temozolomide chemotherapy alone versus radiotherapy alone for malignant astrocytoma in the elderly: the NOA-08 randomised, phase 3 trial. Lancet Oncol 2012;13:707-15. [Crossref] [PubMed]
- Malmström A, Grønberg BH, Marosi C, et al. Temozolomide versus standard 6-week radiotherapy versus hypofractionated radiotherapy in patients older than 60 years with glioblastoma: the Nordic randomised, phase 3 trial. Lancet Oncol 2012;13:916-26. [Crossref] [PubMed]
- Guo C, Yang Q, Xu P, et al. Adjuvant Temozolomide Chemotherapy With or Without Interferon Alfa Among Patients With Newly Diagnosed High-grade Gliomas: A Randomized Clinical Trial. JAMA Netw Open 2023;6:e2253285. [Crossref] [PubMed]
- Weller M, Butowski N, Tran DD, et al. Rindopepimut with temozolomide for patients with newly diagnosed, EGFRvIII-expressing glioblastoma (ACT IV): a randomised, double-blind, international phase 3 trial. Lancet Oncol 2017;18:1373-85. [Crossref] [PubMed]
- Souhami L, Seiferheld W, Brachman D, et al. Randomized comparison of stereotactic radiosurgery followed by conventional radiotherapy with carmustine to conventional radiotherapy with carmustine for patients with glioblastoma multiforme: report of Radiation Therapy Oncology Group 93-05 protocol. Int J Radiat Oncol Biol Phys 2004;60:853-60. [Crossref] [PubMed]
- Laprie A, Noel G, Chaltiel L, et al. Randomized phase III trial of metabolic imaging-guided dose escalation of radio-chemotherapy in patients with newly diagnosed glioblastoma (SPECTRO GLIO trial). Neuro Oncol 2024;26:153-63. [Crossref] [PubMed]
- Brown PD, Chung C, Liu DD, et al. A prospective phase II randomized trial of proton radiotherapy vs intensity-modulated radiotherapy for patients with newly diagnosed glioblastoma. Neuro Oncol 2021;23:1337-47. [Crossref] [PubMed]
- Roa W, Brasher PM, Bauman G, et al. Abbreviated course of radiation therapy in older patients with glioblastoma multiforme: a prospective randomized clinical trial. J Clin Oncol 2004;22:1583-8. [Crossref] [PubMed]
- Perry JR, Laperriere N, O'Callaghan CJ, et al. Short-Course Radiation plus Temozolomide in Elderly Patients with Glioblastoma. N Engl J Med 2017;376:1027-37. [Crossref] [PubMed]
- Stupp R, Hegi ME, Mason WP, et al. Effects of radiotherapy with concomitant and adjuvant temozolomide versus radiotherapy alone on survival in glioblastoma in a randomised phase III study: 5-year analysis of the EORTC-NCIC trial. Lancet Oncol 2009;10:459-66. [Crossref] [PubMed]
- Giladi M, Schneiderman RS, Voloshin T, et al. Mitotic Spindle Disruption by Alternating Electric Fields Leads to Improper Chromosome Segregation and Mitotic Catastrophe in Cancer Cells. Sci Rep 2015;5:18046. [Crossref] [PubMed]
- Jaeckle KA, Eyre HJ, Townsend JJ, et al. Correlation of tumor O6 methylguanine-DNA methyltransferase levels with survival of malignant astrocytoma patients treated with bis-chloroethylnitrosourea: a Southwest Oncology Group study. J Clin Oncol 1998;16:3310-5. [Crossref] [PubMed]
- Hegi ME, Diserens AC, Godard S, et al. Clinical trial substantiates the predictive value of O-6-methylguanine-DNA methyltransferase promoter methylation in glioblastoma patients treated with temozolomide. Clin Cancer Res 2004;10:1871-4. [Crossref] [PubMed]
- Ochs K, Kaina B. Apoptosis induced by DNA damage O6-methylguanine is Bcl-2 and caspase-9/3 regulated and Fas/caspase-8 independent. Cancer Res 2000;60:5815-24. [PubMed]
- Liu L, Markowitz S, Gerson SL. Mismatch repair mutations override alkyltransferase in conferring resistance to temozolomide but not to 1,3-bis(2-chloroethyl)nitrosourea. Cancer Res 1996;56:5375-9. [PubMed]
- Gerson SL. MGMT: its role in cancer aetiology and cancer therapeutics. Nat Rev Cancer 2004;4:296-307. [Crossref] [PubMed]
- Hotta T, Saito Y, Fujita H, et al. O6-alkylguanine-DNA alkyltransferase activity of human malignant glioma and its clinical implications. J Neurooncol 1994;21:135-40. [Crossref] [PubMed]
- Esteller M, Garcia-Foncillas J, Andion E, et al. Inactivation of the DNA-repair gene MGMT and the clinical response of gliomas to alkylating agents. N Engl J Med 2000;343:1350-4. [Crossref] [PubMed]
- Hegi ME, Diserens AC, Gorlia T, et al. MGMT gene silencing and benefit from temozolomide in glioblastoma. N Engl J Med 2005;352:997-1003. [Crossref] [PubMed]
- Buckner JC, Brown LD, Kugler JW, et al. Phase II evaluation of recombinant interferon alpha and BCNU in recurrent glioma. J Neurosurg 1995;82:430-5. [Crossref] [PubMed]
- Shen D, Guo CC, Wang J, et al. Interferon-α/β enhances temozolomide activity against MGMT-positive glioma stem-like cells. Oncol Rep 2015;34:2715-21. [Crossref] [PubMed]
- Taylor TE, Furnari FB, Cavenee WK. Targeting EGFR for treatment of glioblastoma: molecular basis to overcome resistance. Curr Cancer Drug Targets 2012;12:197-209. [Crossref] [PubMed]
- Tortora G, Bianco R, Daniele G, et al. Overcoming resistance to molecularly targeted anticancer therapies: Rational drug combinations based on EGFR and MAPK inhibition for solid tumours and haematologic malignancies. Drug Resist Updat 2007;10:81-100. [Crossref] [PubMed]
- Shinojima N, Tada K, Shiraishi S, et al. Prognostic value of epidermal growth factor receptor in patients with glioblastoma multiforme. Cancer Res 2003;63:6962-70. [PubMed]
- Westphal M, Heese O, Steinbach JP, et al. A randomised, open label phase III trial with nimotuzumab, an anti-epidermal growth factor receptor monoclonal antibody in the treatment of newly diagnosed adult glioblastoma. Eur J Cancer 2015;51:522-32. [Crossref] [PubMed]
- Wygoda Z, Kula D, Bierzyńska-Macyszyn G, et al. Use of monoclonal anti-EGFR antibody in the radioimmunotherapy of malignant gliomas in the context of EGFR expression in grade III and IV tumors. Hybridoma (Larchmt) 2006;25:125-32. [Crossref] [PubMed]
- Gzell C, Back M, Wheeler H, et al. Radiotherapy in Glioblastoma: the Past, the Present and the Future. Clin Oncol (R Coll Radiol) 2017;29:15-25. [Crossref] [PubMed]
- Amelio D, Lorentini S, Schwarz M, et al. Intensity-modulated radiation therapy in newly diagnosed glioblastoma: a systematic review on clinical and technical issues. Radiother Oncol 2010;97:361-9. [Crossref] [PubMed]
- Hermanto U, Frija EK, Lii MJ, et al. Intensity-modulated radiotherapy (IMRT) and conventional three-dimensional conformal radiotherapy for high-grade gliomas: does IMRT increase the integral dose to normal brain? Int J Radiat Oncol Biol Phys 2007;67:1135-44. [Crossref] [PubMed]
- Shaffer R, Nichol AM, Vollans E, et al. A comparison of volumetric modulated arc therapy and conventional intensity-modulated radiotherapy for frontal and temporal high-grade gliomas. Int J Radiat Oncol Biol Phys 2010;76:1177-84. [Crossref] [PubMed]
- Adeberg S, Harrabi SB, Bougatf N, et al. Intensity-modulated proton therapy, volumetric-modulated arc therapy, and 3D conformal radiotherapy in anaplastic astrocytoma and glioblastoma: A dosimetric comparison. Strahlenther Onkol 2016;192:770-9. [Crossref] [PubMed]
- Sheu T, Briere TM, Olanrewaju AM, et al. Intensity Modulated Radiation Therapy Versus Volumetric Arc Radiation Therapy in the Treatment of Glioblastoma-Does Clinical Benefit Follow Dosimetric Advantage? Adv Radiat Oncol 2018;4:50-6. [Crossref] [PubMed]
- Singh R, Lehrer EJ, Wang M, et al. Dose Escalated Radiation Therapy for Glioblastoma Multiforme: An International Systematic Review and Meta-Analysis of 22 Prospective Trials. Int J Radiat Oncol Biol Phys 2021;111:371-84. [Crossref] [PubMed]
- Mori T, Mizumoto M, Maebayashi K, et al. Proton beam therapy for gliomas: a multicenter prospective registry study from all proton beam facilities in Japan. J Radiat Res 2023;64:i59-68. [Crossref]
- Chamberlant I, Eber J, Antoni D, et al. Proton therapy and gliomas: a systematic review. Radiation 2021;1:218-33. [Crossref]
- Niyazi M, Andratschke N, Bendszus M, et al. ESTRO-EANO guideline on target delineation and radiotherapy details for glioblastoma. Radiother Oncol 2023;184:109663. [Crossref] [PubMed]
- Kruser TJ, Bosch WR, Badiyan SN, et al. NRG brain tumor specialists consensus guidelines for glioblastoma contouring. J Neurooncol 2019;143:157-66. [Crossref] [PubMed]
- Gebhardt BJ, Dobelbower MC, Ennis WH, et al. Patterns of failure for glioblastoma multiforme following limited-margin radiation and concurrent temozolomide. Radiat Oncol 2014;9:130. [Crossref] [PubMed]
- McDonald MW, Shu HK, Curran WJ Jr, et al. Pattern of failure after limited margin radiotherapy and temozolomide for glioblastoma. Int J Radiat Oncol Biol Phys 2011;79:130-6. [Crossref] [PubMed]
- Rudra S, Hui C, Rao YJ, et al. Effect of Radiation Treatment Volume Reduction on Lymphopenia in Patients Receiving Chemoradiotherapy for Glioblastoma. Int J Radiat Oncol Biol Phys 2018;101:217-25. [Crossref] [PubMed]
- Patil CG, Yi A, Elramsisy A, et al. Prognosis of patients with multifocal glioblastoma: a case-control study. J Neurosurg 2012;117:705-11. [Crossref] [PubMed]
- Haque W, Thong Y, Verma V, et al. Patterns of management and outcomes of unifocal versus multifocal glioblastoma. J Clin Neurosci 2020;74:155-9. [Crossref] [PubMed]
- Whittle IR, Denholm SW, Gregor A. Management of patients aged over 60 years with supratentorial glioma: lessons from an audit. Surg Neurol 1991;36:106-11. [Crossref] [PubMed]
- Haque W, Verma V, Butler EB, et al. Addition of chemotherapy to hypofractionated radiotherapy for glioblastoma: practice patterns, outcomes, and predictors of survival. J Neurooncol 2018;136:307-15. [Crossref] [PubMed]
- Gondi V, Pugh S, Tsien C, et al. Radiotherapy (RT) Dose-intensification (DI) Using Intensity-modulated RT (IMRT) versus Standard-dose (SD) RT with Temozolomide (TMZ) in Newly Diagnosed Glioblastoma (GBM): Preliminary Results of NRG Oncology BN001. Int J Radiat Oncol Biol Phys 2020;108:S22-3. [Crossref]
- Shi W, Kleinberg L, Jeyapalan SA, et al. Phase III TRIDENT trial: Radiation and temozolomide +/- tumor treating fields in newly diagnosed glioblastoma. J Clin Oncol 2020;38:TPS2580. [Crossref]
- Lipkova J, Angelikopoulos P, Wu S, et al. Personalized Radiotherapy Design for Glioblastoma: Integrating Mathematical Tumor Models, Multimodal Scans, and Bayesian Inference. IEEE Trans Med Imaging 2019;38:1875-84. [Crossref] [PubMed]
- Peeken JC, Molina-Romero M, Diehl C, et al. Deep learning derived tumor infiltration maps for personalized target definition in Glioblastoma radiotherapy. Radiother Oncol 2019;138:166-72. [Crossref] [PubMed]
- Arabzadeh A, Mortezazadeh T, Aryafar T, et al. Therapeutic potentials of resveratrol in combination with radiotherapy and chemotherapy during glioblastoma treatment: a mechanistic review. Cancer Cell Int 2021;21:391. [Crossref] [PubMed]
- Choi J, Kim G, Cho SB, et al. Radiosensitizing high-Z metal nanoparticles for enhanced radiotherapy of glioblastoma multiforme. J Nanobiotechnology 2020;18:122. [Crossref] [PubMed]